Chapter 7: Development of the Brain and Nervous System
7.6: Trade-off between plasticity and efficiency
When considering neuroplasticity, it’s important to recognize that different brain networks develop at different timescales (Dow-Edwards et al., 2019). For example, as mentioned earlier, brain regions involved in higher-order cognition, such as self-regulation, undergo a protracted, late development—neuroplasticity during adolescence is geared more toward refining those higher-order executive functions than basic sensory processes. The neural circuitry underlying basic sensory processes has peak plasticity during infancy and is firmly established by early childhood. It would be inefficient for the adolescent or adult brain to constantly re-learn how to process sensory information. This example highlights the trade-off between neuroplasticity and neural efficiency.
Neural efficiency refers to the process of the brain expending fewer energy resources to meet task demands. This efficiency can also be represented in how easily different brain regions are able to communicate with each other. Indeed, research has shown that the efficiency of information flow across the brain is accelerated during adolescence because axons become more insulated with myelin, which improves the speed of electrical transmission and reduces the energy needed to carry out this process (Spear, 2013). However, neural efficiency emerges separately in different brain regions depending on developmental timescales. For example, sensory processing and its underlying circuitry develop rapidly during early childhood and become more efficient and less malleable by late childhood.
One important factor underlying differences in neural efficiency is the formation of synapses. Early research showed a pattern in synapse formation during brain development such that there is first a rapid overproduction of synapses, followed by a programmed elimination of non-functional synapses, which eventually brings the overall number of synapses down to adult levels (Huttenlocher, 1979; Huttenlocher & Dabholkar, 1997; Shonkoff & Phillips, 2000). This synapse-formation pattern emerges at different timescales for different brain networks—synapse formation related to sensory processes peaks first, followed by language-related processes, and finally higher-order cognitive functions (Figure 6). Relevant to neural efficiency, one hypothesis proposes that excess synapses during adolescence in frontal lobe regions, such as the prefrontal cortex, may render information processing less efficient in those brain regions (Blakemore, 2012). In other words, during adolescence the brain may require many synapses to carry out cognitive processes. However, as these neuronal connections and functional networks become refined throughout development, the brain may require fewer synapses to carry out the same cognitive processes. In this way, the brain is hypothesized to shift toward prioritizing the most efficient synapses, which may ultimately lead to more efficient cognitive processing.
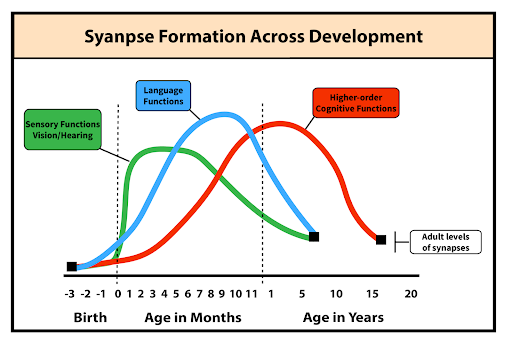
The tradeoff between neuroplasticity and stable efficient processing can be illustrated by recent research. In one study, a drug (valproate) was shown to reopen a critical period for a sensory task—when given this drug, adults could better learn to identify the names of musical pitches (termed “absolute pitch”), a skill that typically can only be acquired early in life (Gervain et al., 2013). While this finding has exciting ramifications for increasing plasticity in adults to learn new skills and rewire undesired established neural pathways (e.g., for treating addiction or psychiatric disorders), one must exercise caution when tampering with plasticity in the brain. For example, critical periods have evolved for a reason, and reopening critical periods might destabilize long-established neural circuits for efficiently processing things like how to see the world or understand language.
Finally, psychedelic drugs have been recognized for their potential to treat addiction and psychological disorders. A recent study suggests that psychedelic drugs can reopen critical periods and increase plasticity in the brain in mice (Nardou et al., 2023). Additionally, psychedelics may induce cellular and molecular adaptations related to neuroplasticity and these may support the clinical effects of psychedelics in humans (de Vos et al., 2021; Calder & Hassler, 2023). While more work on psychedelics and neuroplasticity is needed, the possibility of inducing neuroplasticity could lead to rich learning and brain restructuring, but it also underscores the importance of working with a trained professional when the brain is in a more malleable state so as to not destabilize desired neural circuits.
Media Attributions
- Syanpse Formation Across Development © National Library of Medicine is licensed under a CC BY-NC-SA (Attribution NonCommercial ShareAlike) license
The process in which the brain spends fewer energy resources to carry out specific brain functions and subsequent behavior. Typically indicative of a matured functional brain network.