Energy and Metabolism
Section Goals
By the end of this section, you will be able to do the following:
- Explain metabolic pathways and describe the two major types
- Discuss how chemical reactions play a role in energy transfer
- Describe how cells store and transfer free energy using ATP
Virtually every task performed by living organisms requires energy. Organisms require energy to perform heavy labor and exercise, but humans also use considerable energy while thinking and even during sleep. Every organism’s living cells constantly use energy. Organisms import nutrients and other molecules. They metabolize (break down) and may be synthesized into new molecules. If necessary, molecules modify, move around the cell, and may distribute themselves to the entire organism. For example, the large proteins that make up muscles are actively built from smaller molecules. Complex carbohydrates break down into simple sugars that the cell uses for energy. Just as energy is required to both build and demolish a building, energy is required to synthesize and break down molecules. Additionally, signaling molecules such as hormones and neurotransmitters transport between cells. Cells ingest and break down bacteria and viruses. Cells must also export waste and toxins to stay healthy, and many cells must swim or move surrounding materials via the beating motion of cellular appendages like cilia and flagella.
The cellular processes that we listed above require a steady supply of energy. From where and in what form, does this energy come? How do living cells obtain energy, and how do they use it? Fortunately for us, our cells—and those of other living organisms—are excellent at harvesting energy from glucose and other organic molecules, such as fats and amino acids. Here, we’ll go through an overview of how cells use energy and replenish it.
Scientists use the term bioenergetics to discuss the concept of energy flow (Figure 1) through living systems, such as cells. Cellular processes such as building and breaking down complex molecules occur through stepwise chemical reactions. Some of these chemical reactions are spontaneous and release energy, whereas others require energy to proceed. Just as living things must continually consume food to replenish what they have used, cells must continually obtain more energy to replenish that which the many energy-requiring chemical reactions that constantly take place use. All of the chemical reactions that transpire inside cells, including those that use and release energy, are the cell’s metabolism.
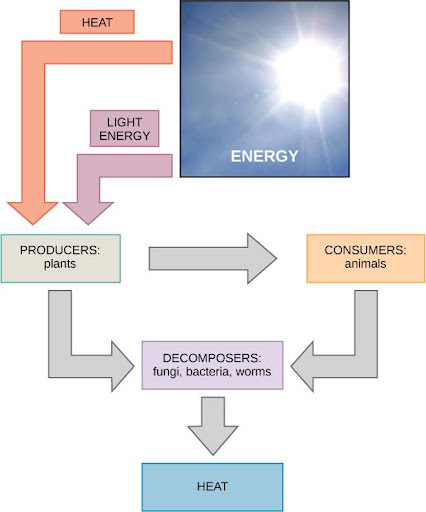
Carbohydrate Metabolism
Sugar (a simple carbohydrate) metabolism (chemical reactions) is a classic example of the many cellular processes that use and produce energy. Living things consume sugar as a major energy source because sugar molecules have considerable energy stored within their bonds. The following equation describes the breakdown of glucose, a simple sugar:
C6H12O6 + 6O2 → 6CO2 + 6H2O + energy
Consumed carbohydrates have their origins in photosynthesizing organisms like plants (Figure 2). During photosynthesis, plants use the energy of sunlight to convert carbon dioxide gas (CO2) into sugar molecules, like glucose (C6H12O6). Because this process involves synthesizing a larger, energy-storing molecule, it requires an energy input to proceed. The following equation (notice that it is the reverse of the previous equation) describes the synthesis of glucose:
6CO2 + 6H2O + energy → C6H12O6 + 6O2.
During the chemical reactions in the process of photosynthesis, energy is in the form of a very high-energy molecule scientists call ATP, or adenosine triphosphate. ATP is the primary energy currency of all cells. Just as the dollar is the currency we use to buy goods, cells use ATP molecules as energy currency to perform immediate work. The sugar (glucose) is stored as starch or glycogen. Energy-storing polymers like these break down into glucose to supply ATP molecules.
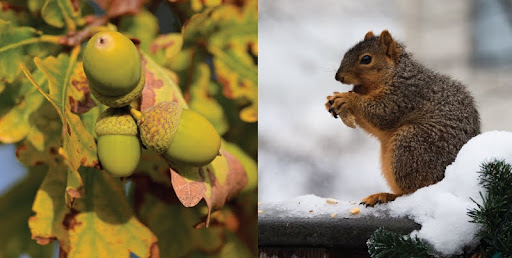
Solar energy is required to synthesize a glucose molecule during the photosynthesis reactions. In photosynthesis, light energy from the sun initially transforms into chemical energy that temporarily stores itself in the energy carrier molecules ATP and NADPH (nicotinamide adenine dinucleotide phosphate). Photosynthesis later uses the stored energy in ATP and NADPH to build one glucose molecule from six molecules of CO2. This process is analogous to eating breakfast in the morning to acquire energy for your body that you can use later in the day. Under ideal conditions, energy from 18 molecules of ATP is required to synthesize one glucose molecule during photosynthesis reactions. Glucose molecules can also combine with and convert into other sugar types. When an organism consumes sugars, glucose molecules eventually make their way into each organism’s living cell. Inside the cell, each sugar molecule breaks down through a complex series of chemical reactions. The goal of these reactions is to harvest the energy stored inside the sugar molecules. The harvested energy makes high-energy ATP molecules, which perform work, powering many chemical reactions in the cell. The amount of energy needed to make one glucose molecule from six carbon dioxide molecules is 18 ATP molecules and 12 NADPH molecules (each one of which is energetically equivalent to three ATP molecules), or a total of 54 molecule equivalents required for synthesizing one glucose molecule. This process is a fundamental and efficient way for cells to generate the molecular energy that they require.
Metabolic Pathways
The processes of making and breaking down sugar molecules illustrate two types of metabolic pathways. A metabolic pathway is a series of interconnected biochemical reactions that convert a substrate molecule or molecules, step-by-step, through a series of metabolic intermediates, eventually yielding a final product or products.
In the case of sugar metabolism, the first metabolic pathway synthesizes sugar from smaller molecules, and the other pathway breaks sugar down into smaller molecules. Scientists call these two opposite processes—the first requiring energy and the second producing energy—anabolic (building) and catabolic (breaking down) pathways, respectively (Figure 3).
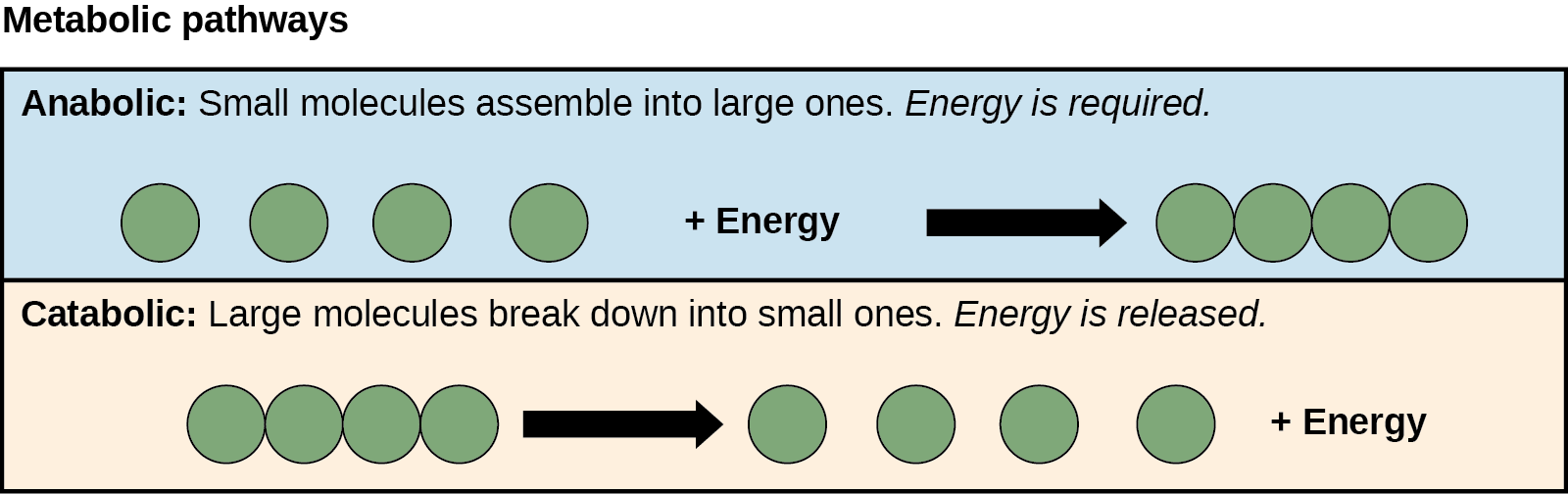
The reactions that allow energy to be extracted from molecules such as glucose, fats, and amino acids are catabolic because they involve breaking a larger molecule into smaller pieces. For example, when glucose is broken down in the presence of oxygen, it’s converted into six carbon dioxide molecules and six water molecules:
C6H12O6 + 6O2 → 6CO2 + 6H2O
The above reaction, as written, is simply a combustion reaction, similar to what takes place when you burn a piece of wood in a fireplace or gasoline in an engine. Does this mean that glucose is continually combusting inside your cells? Thankfully, not quite! The combustion reaction describes the overall process that takes place, but inside the cell, this process is broken down into many smaller steps. The energy contained in the bonds of glucose is released in small bursts, and some of it can be captured in the form of adenosine triphosphate (ATP), a small molecule that is used to power reactions in the cell. Much of the energy from glucose is still lost as heat, but enough is captured to keep the metabolism of the cell running.
As a glucose molecule is gradually broken down, some of the breakdown steps release energy that is captured directly as ATP. In these steps, a phosphate group is transferred from a pathway intermediate straight to ADP, a process known as substrate-level phosphorylation. Many more steps, however, indirectly produce ATP. In these steps, electrons from glucose are transferred to small molecules known as electron carriers. The electron carriers take the electrons to a group of proteins in the inner membrane of the mitochondrion, called the electron transport chain. As electrons move through the electron transport chain, they go from a higher to a lower energy level and are ultimately passed to oxygen (forming water). The energy released in the electron transport chain is captured as a proton gradient, which powers the production of ATP by a membrane protein called ATP synthase. This process is known as oxidative phosphorylation. A simplified diagram of oxidative and substrate-level phosphorylation is shown below.
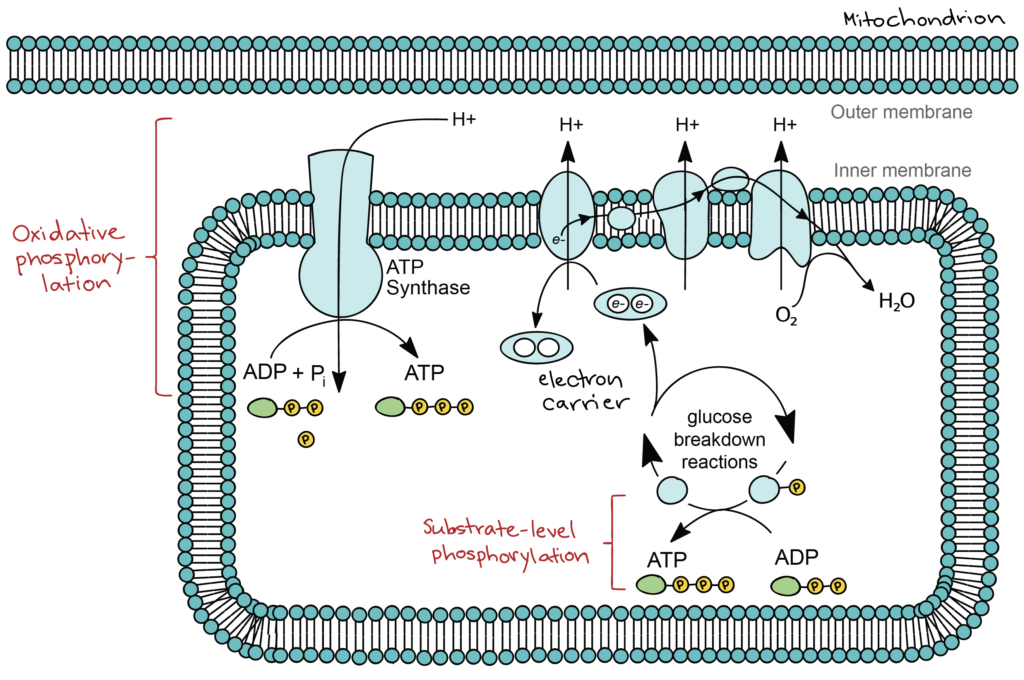
When organic fuels like glucose are broken down using an electron transport chain that ends with oxygen, the breakdown process is known as aerobic respiration (aerobic = oxygen-requiring). Most eukaryotic cells, as well as many bacteria and other prokaryotes, can carry out aerobic respiration. Some prokaryotes have pathways similar to aerobic respiration but with a different inorganic molecule, such as sulfur, substituted for oxygen. These pathways are not oxygen-dependent, so the breakdown process is called anaerobic respiration (anaerobic = non-oxygen-requiring). Officially, both processes are examples of cellular respiration, the breakdown of organic fuels using an electron transport chain. However, cellular respiration is commonly used as a synonym for aerobic respiration.
ATP in Living Systems
A living cell cannot store significant amounts of free energy. Excess free energy would result in an increase of heat in the cell, which would result in excessive thermal motion that could damage and then destroy the cell. Rather, a cell must be able to handle that energy in a way that enables the cell to store energy safely and release it for use only as needed. Living cells accomplish this by using the compound adenosine triphosphate (ATP). ATP is often called the “energy currency” of the cell, and, like currency, this versatile compound can be used to fill any energy need of the cell. How? It functions similarly to a rechargeable battery.
When ATP is broken down, usually by the removal of its terminal phosphate group, energy is released. The energy is used to do work by the cell, usually by the released phosphate binding to another molecule, activating it. For example, in the mechanical work of muscle contraction, ATP supplies the energy to move the contractile muscle proteins. Recall the active transport work of the sodium-potassium pump in cell membranes. ATP alters the structure of the integral protein that functions as the pump, changing its affinity for sodium and potassium. In this way, the cell performs work, pumping ions against their electrochemical gradients.
ATP Structure and Function
At the heart of ATP is a molecule of adenosine monophosphate (AMP), which is composed of an adenine molecule bonded to a ribose molecule and a single phosphate group (Figure 5).
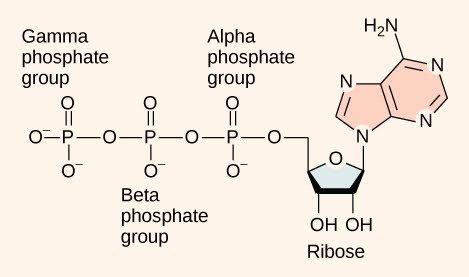
Ribose is a five-carbon sugar found in RNA, and AMP is one of the nucleotides in RNA. The addition of a second phosphate group to this core molecule results in the formation of adenosine diphosphate (ADP); the addition of a third phosphate group forms adenosine triphosphate (ATP). The addition of a phosphate group to a molecule requires energy. Phosphate groups are negatively charged and thus repel one another when they are arranged in series, as they are in ADP and ATP. This repulsion makes the ADP and ATP molecules inherently unstable. The release of one or two phosphate groups from ATP, a process called dephosphorylation, releases energy.
Energy from ATP
Hydrolysis is the process of breaking complex macromolecules apart. During hydrolysis, water is split or lysed, and the resulting hydrogen atom (H+) and a hydroxyl group (OH–) are added to the larger molecule. The hydrolysis of ATP produces ADP, together with an inorganic phosphate ion (Pi), and the release of free energy. To carry out life processes, ATP is continuously broken down into ADP, and like a rechargeable battery, ADP is continuously regenerated into ATP by the reattachment of a third phosphate group. Water, which was broken down into its hydrogen atom and hydroxyl group during ATP hydrolysis, is regenerated when a third phosphate is added to the ADP molecule, reforming ATP.
Obviously, energy must be infused into the system to regenerate ATP. Where does this energy come from? In nearly every living thing on earth, the energy comes from the metabolism of glucose. In this way, ATP is a direct link between the limited set of exergonic pathways of glucose catabolism and the multitude of endergonic pathways that power living cells.
Phosphorylation
Recall that, in some chemical reactions, enzymes may bind to several substrates that react with each other on the enzyme, forming an intermediate complex. An intermediate complex is a temporary structure, and it allows one of the substrates (such as ATP) and reactants to react with each other more readily; in reactions involving ATP, ATP is one of the substrates, and ADP is a product. During an endergonic chemical reaction, ATP forms an intermediate complex with the substrate and enzyme in the reaction. This intermediate complex allows the ATP to transfer its third phosphate group, with its energy, to the substrate, a process called phosphorylation. Phosphorylation refers to the addition of the phosphate (~P). This process is illustrated by the following generic reaction:
A + enzyme + ATP → [A − enzyme − ~P] → B + enzyme + ADP + phosphate ion
When the intermediate complex breaks apart, the energy is used to modify the substrate and convert it into a reaction product. The ADP molecule and a free phosphate ion are released into the medium and are available for recycling through cell metabolism.
Substrate Phosphorylation
ATP is generated through two mechanisms during the breakdown of glucose. A few ATP molecules are generated (that is, regenerated from ADP) as a direct result of the chemical reactions that occur in the catabolic pathways. A phosphate group is removed from an intermediate reactant in the pathway, and the free energy of the reaction is used to add the third phosphate to an available ADP molecule, producing ATP (Figure 6). This very direct method of phosphorylation is called substrate-level phosphorylation.
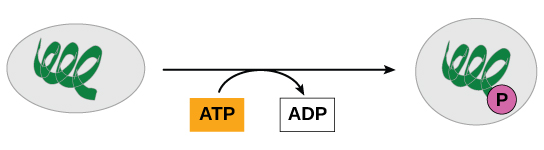
Oxidative Phosphorylation
Most of the ATP generated during glucose catabolism, however, is derived from a much more complex process, chemiosmosis, which takes place in mitochondria (Figure 7) within a eukaryotic cell or the plasma membrane of a prokaryotic cell.
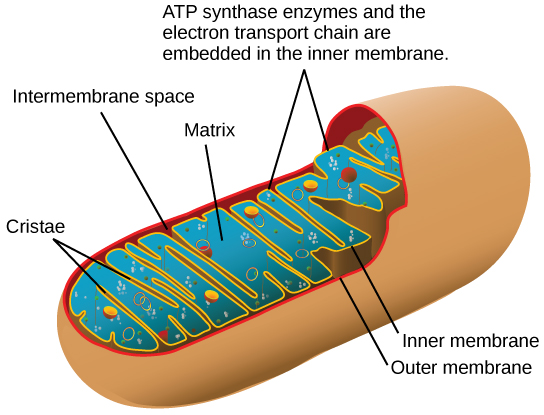
Chemiosmosis, a process of ATP production in cellular metabolism, generates 90 percent of the ATP made during glucose catabolism. It is also the method used in the light reactions of photosynthesis to harness the energy of sunlight. The production of ATP using the process of chemiosmosis is called oxidative phosphorylation because oxygen is involved in the process.
Did I Get It?
Cells perform the functions of life through various chemical reactions. A cell’s metabolism refers to the chemical reactions that take place within it. There are metabolic reactions that involve breaking down complex chemicals into simpler ones, such as breaking down large macromolecules. Scientists refer to this process as catabolism, and we associate such reactions with an energy release. On the other end of the spectrum, anabolism refers to metabolic processes that build complex molecules out of simpler ones, such as macromolecule synthesis. Anabolic processes require energy. Glucose synthesis and glucose breakdown are examples of anabolic and catabolic pathways, respectively.
ATP functions as the energy currency for cells. It allows the cell to store energy briefly and transport it within the cell to support endergonic chemical reactions. The structure of ATP is that of an RNA nucleotide with three phosphates attached. As ATP is used for energy, a phosphate group or two is detached, and either ADP or AMP is produced. Energy derived from glucose catabolism is used to convert ADP into ATP. When ATP is used in a reaction, the third phosphate is temporarily attached to a substrate in a process called phosphorylation. The two processes of ATP regeneration that are used in conjunction with glucose catabolism are substrate-level phosphorylation and oxidative phosphorylation through the process of chemiosmosis.
CC Licensed Content, Shared Previously
- Biology 2e. Authors: Mary Ann Clark, Matthew Douglas and Jung Choi. Provided by: OpenStax CNX. Located at: Biology 2e. License: CC BY: Attribution 4.0.
- Biology for Majors I. Author: Shelly Carter. Provided by: Lumen Learning. Located at: Biology for Majors I | Simple Book Production. License: CC BY: Attribution 4.0.
- Introduction to cellular respiration and redox. Provided by: Khan Academy. Located at: Introduction to Cellular Respiration and Redox. License: CC BY-NC-SA 4.0