Animal Bioenergetics
Section Goals
By the end of this section, you will be able to do the following:
- Explain the difference between endotherms and ectotherms.
- Interpret graphs related to endotherms and ectotherms.
- Relate bioenergetics to body size, levels of activity, and the environment
- Describe thermoregulation of endothermic and ectothermic animals
Endotherms and Ectotherms
What’s it like outside today? If it’s winter where you are, it might be pretty cold. If it’s summer, it might be pretty hot. Either way, odds are that your core body temperature is right around 98.6℉/37℃. Mechanisms like shivering and sweating kick in when your body gets too cold or too hot, keeping your internal temperature steady.
Not all organisms keep their body temperature in as narrow a range as we humans do, but virtually every animal on the planet has to regulate body temperature to some degree—if only to keep the water in its cells from turning to ice or to avoid denaturing its metabolic enzymes with heat.
Broadly speaking, animals can be divided into two groups based on how they regulate body temperature: endotherms and ectotherms. Let’s take a closer look at the difference between these two groups.
People, polar bears, penguins, and prairie dogs, like most other birds and mammals, are endotherms. Iguanas and rattlesnakes, like most other reptiles—along with most fishes, amphibians, and invertebrates—are ectotherms.
Endotherms generate most of the heat they need internally. When it’s cold out, they increase metabolic heat production to keep their body temperature constant. Because of this, the internal body temperature of an endotherm is more or less independent of the temperature of the environment.
The sum total of the biochemical reactions that take place in an organism are called its metabolism. Metabolic reactions involve breaking down fuel molecules, such as sugars, and using the energy stored in them to do work. The processes that convert energy stored in food molecules into biological work are not very efficient, so heat is generated as a byproduct. The higher an organism’s metabolic rate—the amount of chemical fuel it burns in a given period of time—the more heat it will produce.
So, as an endotherm is exposed to colder external temperatures, it will increase its metabolic rate, burn more fuel, and produce extra heat to keep its body temperature constant. This pattern is shown in Figure 1 below: the mouse maintains a steady body temperature close to 37℃ across a wide range of external temperatures.
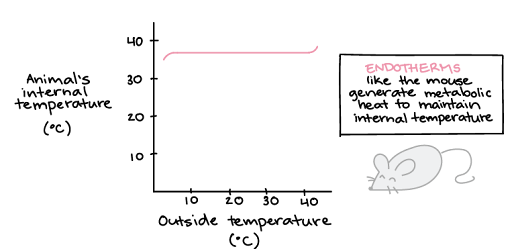
For ectotherms, on the other hand, body temperature mainly depends on external heat sources. That is, ectotherm body temperature rises and falls along with the temperature of the surrounding environment (Figure 2). Although ectotherms do generate some metabolic heat—like all living things—ectotherms can’t increase this heat production to maintain a specific internal temperature.
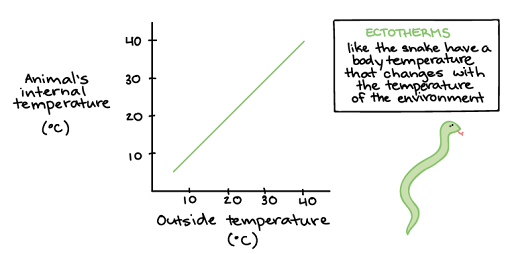
Most ectotherms do regulate their body temperature to some degree, though. They just don’t do it by producing heat. Instead, they use other strategies, such as behavior—seeking sun, shade, etc.—to find environments whose temperature meets their needs.
Some species blur the line between endotherms and ectotherms. Animals that hibernate, for instance, are endothermic when they are active but resemble ectotherms when they are hibernating. Large fish like tuna and sharks generate and conserve enough heat to raise their body temperature above that of the surrounding water. Still, unlike a true endotherm, they don’t maintain a specific body temperature. Even some insects can use metabolic heat to increase body temperature by contracting their flight muscles!
One other important point: as a general rule, endotherms have considerably higher metabolic rates than ectotherms. That’s because they have to burn large quantities of fuel—food—to maintain their internal body temperature.
Why regulate temperature?
There are some basic limits on survivable body temperature for most animals. At one end of the spectrum, water freezes at 32℉/0℃ to form ice. If ice crystals form inside a cell, they’ll generally rupture its membranes. At the other end of the spectrum, enzymes and other proteins in cells often start to lose shape and function, or denature, at temperatures above 104℉/40℃.
Why do many organisms—including you and me—keep their body temperature in a narrower range than this? The rate of chemical reactions changes with temperature, both because temperature affects the rate of collisions between molecules and because the enzymes that control the reactions may be temperature-sensitive. Reactions tend to go faster with higher temperatures, up to a point beyond which their rate drops sharply as their enzymes denature.
Each species has its network of metabolic reactions and set of enzymes optimized for a particular temperature range. By keeping body temperature in that target range, organisms ensure that their metabolic reactions run properly.
Temperature balance
For both endotherms and ectotherms, body temperature depends on the balance between heat generated by the organism and heat exchanged with—lost to or gained from—the environment. Heat always moves from warmer to cooler objects, as described in the Second Law of Thermodynamics. There are three main ways that an organism can exchange heat with its environment: radiation, conduction—along with convection—and evaporation.
- Radiation: Radiation is the transfer of heat from a warmer object to a cooler one by infrared radiation, that is, without direct contact. You’ve experienced radiation if you’ve been warmed by heat from the sun, a fire, or a radiator in a building.
- Conduction: Heat can be transferred between two objects in direct contact by means of conduction. If you pick up an ice cube, you’ll lose heat to the ice by means of conduction. If you walk barefoot on stone on a sunny day, on the other hand, you’ll absorb heat from the stone by conduction.
- Evaporation: Vaporization of water from a surface leads to loss of heat—for example, when sweat evaporates from your skin.
Did I Get It?
Hint/Explanation
We can use what we know about how endotherms and ectotherms maintain body temperature to figure out which line corresponds to which animal.
To start with, let’s “translate” the Y axis of the graph. Oxygen consumption is a common measure of metabolic rate because O2 gas is used up when fuel molecules are broken down during cellular respiration. The faster an organism is using up oxygen, the higher its metabolic rate. We could measure CO2 production or heat production to determine metabolic rate.
So, which curve represents the endotherm? Endotherms increase their metabolic rate as temperature drops, producing more heat and thus keeping their internal temperature up. The only curve that goes up as temperature goes down is the upper curve— the blue curve A— so this must be the endotherm (Figure 4).
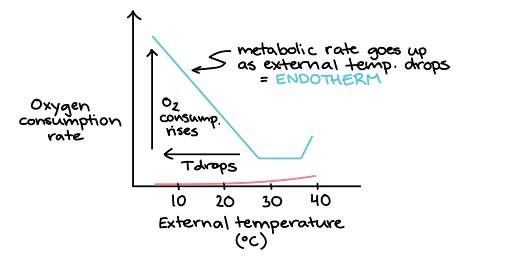
The flat part of the curve corresponds to the thermoneutral zone—the range of external temperatures over which the endotherm doesn’t have to expend extra energy above its basal, or resting, metabolic rate to maintain body temperature. The increase in metabolic rate at higher temperatures represents the expenditure of energy to try to cool the body and/or the heating of tissue as cooling systems fail.
The remaining curve— the red curve B— must be our ectotherm (Figure 5). Not only is the ectotherm’s metabolic rate consistently lower than that of the endotherm, but it also drops as external temperature decreases—the opposite pattern from the endotherm. That’s because biochemical reactions tend to slow down at low temperatures, such as those of an ectotherm’s body when external temperature decreases.
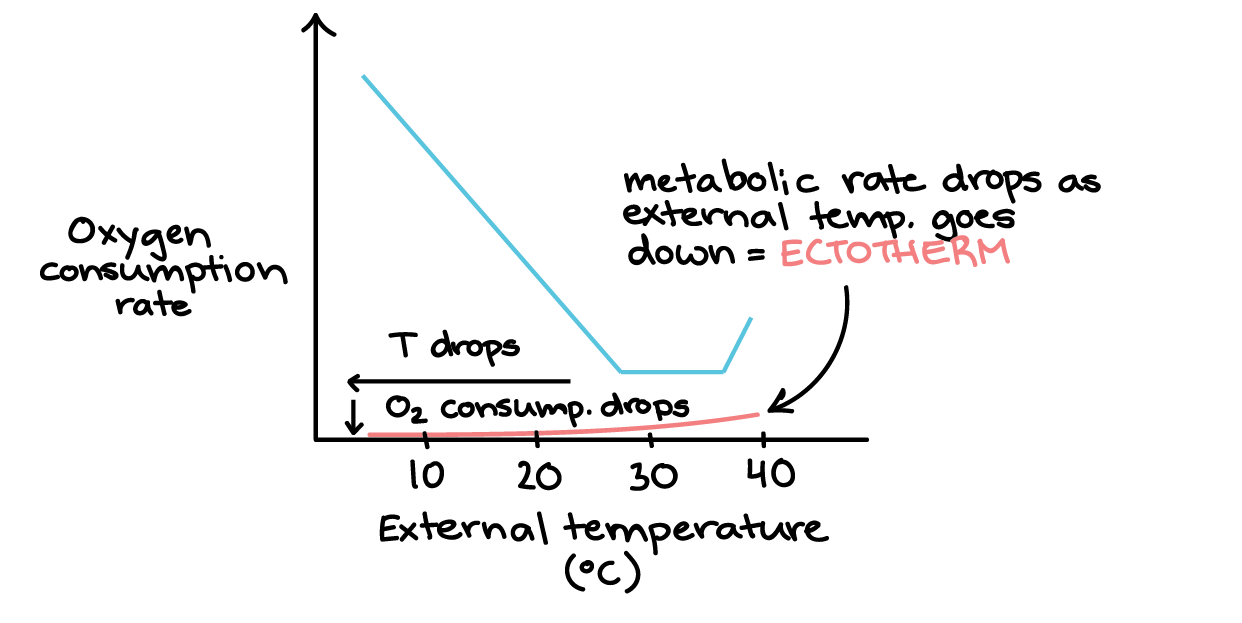
Metabolic Rate
You may be used to thinking about metabolism in terms of human eating patterns. For instance, a person who has to eat constantly to keep from losing weight may say they have a “fast metabolism,” while a person who eats only a little and still gains weight may say they have a “slow metabolism.”
However, metabolism isn’t unique to humans. In fact, when you get right down to it, metabolism just refers to the sum total of the biochemical reactions that take place in an organism’s body. So, every living thing has a metabolism, from a bacterium to a plant to you!
What, exactly, is the rate of an organism’s metabolism? Broadly speaking, metabolic rate refers to how quickly fuels (such as sugars) are broken down to keep the organism’s cells running. There are general differences in metabolic rate among species, and the environmental conditions and activity level of an individual organism will also affect its metabolic rate.
Metabolism and heat production
It’s probably not news to you that animals (such as humans) need food as a source of energy. But why is this the case?
The molecules in your breakfast, lunch, or dinner have energy stored in their chemical bonds. Some of your body’s metabolic reactions, like the ones that make up cellular respiration, extract this energy and capture part of it as adenosine triphosphate (ATP) (Figure 6). This energy-carrying molecule can, in turn, be used to power other metabolic reactions that keep your cells running.
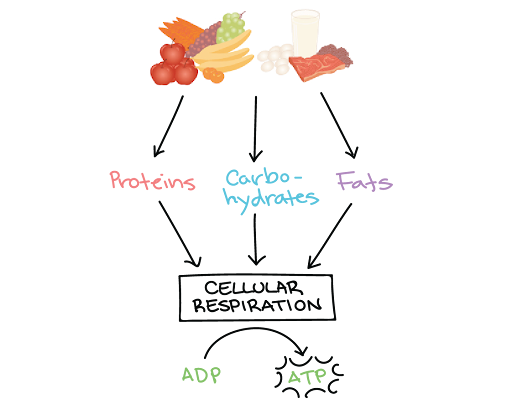
Molecules from food are also used as building blocks for the structures of your body. For instance, proteins from your food are broken down into their parts (amino acids) and may be used to build new proteins in your cells. If you eat more than enough food to replenish the energy you use, food energy may also be stored as glycogen (a chain of linked glucose molecules) or as triglycerides (fat molecules) for later use.
The business of extracting energy from fuel molecules and using it to power cellular reactions is a process that could be more efficient. In fact, no energy transfer can be perfectly efficient – that’s a basic law of physics. Instead, each time energy changes forms, some amount of it is converted into a non-usable form. In the reactions of an animal’s metabolism, much of the energy stored in fuel molecules is released as heat.
This release of heat is a good thing! Some animals can use (and regulate) their metabolic heat production to maintain a relatively constant body temperature. These animals, called endotherms, include mammals, such as humans, as well as birds. Ectotherms, on the other hand, are animals that don’t use metabolic heat production to maintain a constant body temperature. Instead, their body temperature changes with the temperature of the environment. Lizards and snakes are examples of ectotherms (Figure 7).
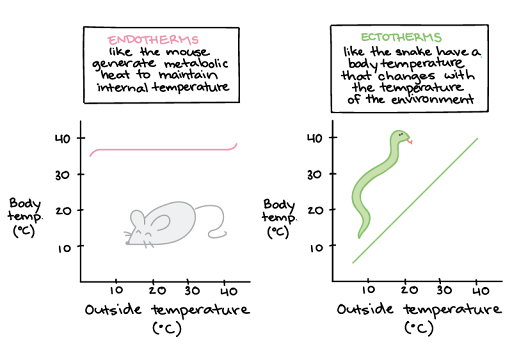
Metabolic rate
The amount of energy expended by an animal over a specific period of time is called its metabolic rate. Metabolic rate may be measured in joules, calories, or kilocalories per unit of time. You may also see metabolic rate given as oxygen consumed (or carbon dioxide produced) per unit time. Oxygen is used up in cellular respiration, and carbon dioxide is produced as a by-product, so both of these measurements indicate how much fuel is being burned.
In some cases, metabolic rate is given for the entire animal. In other cases, metabolic rate is given on a per-mass basis – for example, how much energy one gram of the animal’s tissues uses per unit of time. Per-mass metabolic rates help us make meaningful comparisons between organisms of different sizes.
The “baseline” metabolic rate of an animal is measured as the basal metabolic rate (BMR) for an endotherm or as the standard metabolic rate (SMR) for an ectotherm. Both the BMR and SMR are measures of metabolic rate in animals that are at rest, calm/unstressed, and not actively digesting food (fasting).
- For an endotherm, the BMR is also measured when the animal is in a thermoneutral environment, that is, one where the organism does not expend extra energy (above baseline) to maintain temperature.
- For an ectotherm, SMR will vary with temperature, so any SMR measurement is specific to the temperature at which it’s taken.
Endotherms tend to have basal high metabolic rates and high energy needs, thanks to their maintenance of a constant body temperature. Ectotherms of similar size tend to have much lower standard metabolic rates and energy requirements, sometimes 10% or less of those of comparable endotherms. What about humans? Human adult males typically have a BMR of 1600 to 1800 kcal/day, and human adult females typically have a BMR of 1300 to 1500 kcal/day. That doesn’t mean that’s all the calories you should eat, though! Most people have a higher metabolic rate than this just from carrying out daily activities like standing up, walking around, and working or studying.
Energy requirements related to body size
Which one has a higher basal metabolic rate: a mouse or an elephant? If we look at the metabolic rate of the entire organism, the elephant is going to win – there is way more metabolizing tissue in an elephant than in a mouse. If we look at the per-mass metabolic rate, however, the situation flips. A gram of mouse tissue metabolizes more than ten times faster than a gram of elephant tissue (Figure 8)!
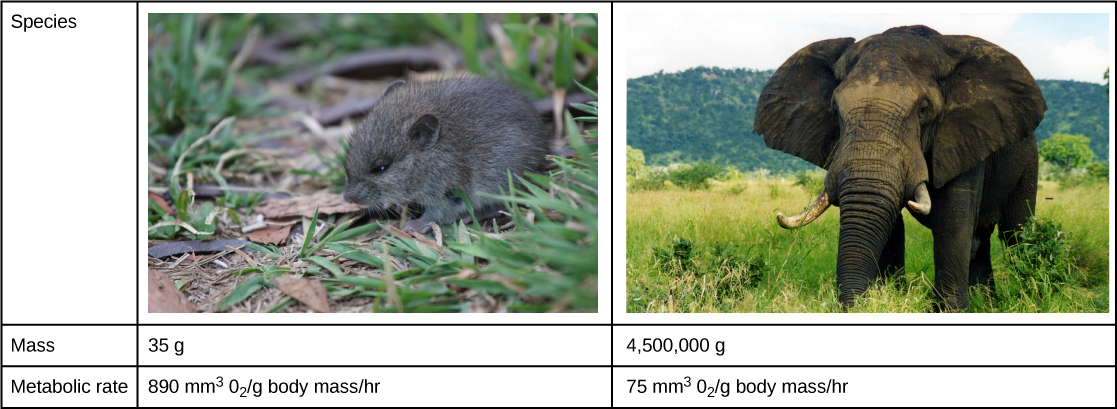
Curiously enough, this is a very general relationship in nature. Among endotherms (animals that use body heat to maintain a constant internal temperature), the smaller the organism’s mass, the higher its basal metabolic rate is likely to be. The relationship between mass and metabolic rate holds true across many species, and even follows a specific mathematical equation.
Why is this the case? The short answer is that we don’t know for sure! Part of the explanation may relate to animals’ surface area-to-volume ratio and how it varies with size. Just as a small cell has more surface area relative to its volume than a large cell, so a small animal has more body surface relative to its volume of metabolizing tissue.
Since animals exchange heat with their environment across their body surfaces, small animals will tend to lose heat to a cooler environment faster than large animals. Because of this, a smaller animal would need more energy and a higher metabolic rate to maintain a constant internal temperature (in an environment below its body temperature).
However, this probably isn’t the full explanation for the relationship between body mass and metabolic rate. Why not? For one thing, the metabolic rates of ectotherms also tend to scale with body mass just like those of endotherms. This is difficult to explain with relation to heat retention and heat loss, since ectotherms don’t maintain a body temperature different from their environment. The real cause of the relationship between metabolic rate and body mass remains an unsolved mystery.
Energy requirements related to levels of activity
The basal metabolic rate (BMR) or standard metabolic rate (SMR) is a measure of an animal’s metabolic rate when it is quiet, not stressed out or excited, and not doing anything active. I don’t know about you, but most of the time, that doesn’t describe me!
The more active an animal is, the more energy must be expended to maintain that activity, and the higher its metabolic rate. For instance, the hamster running on its wheel in Figure 9 below would have a higher metabolic rate than a similar hamster snoozing in the corner.
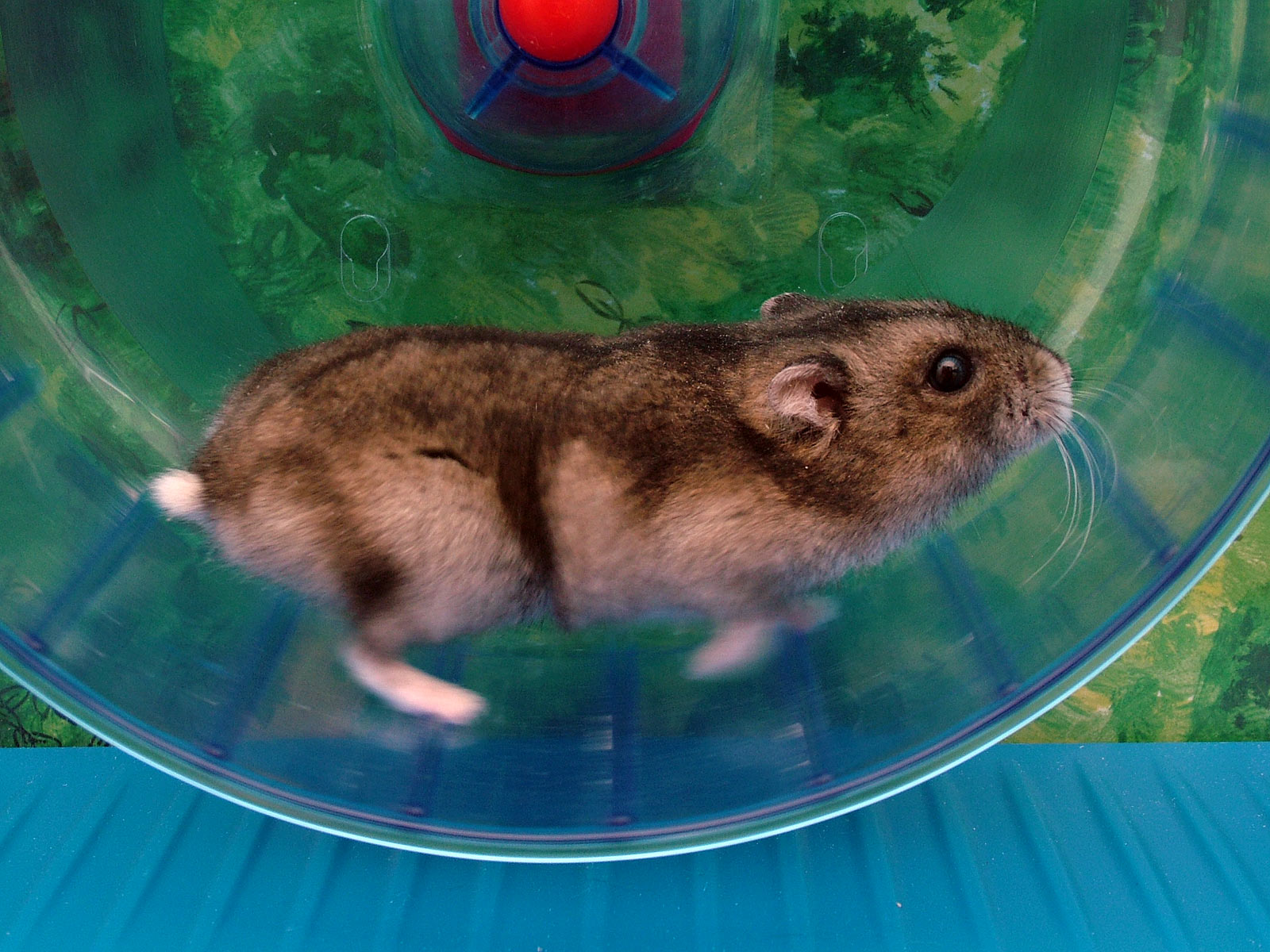
This is something we humans are familiar with from everyday life. For example, if you spend your day going for a long hike or playing sports with friends, you are likely to get pretty hungry (reflecting that you’ve used up a lot of energy and need more fuel). If, on the other hand, you lie in bed all day reading or watching TV, you’ll likely be less hungry because you’ve used up less energy.
For a typical animal, the average daily rate of energy consumption is much higher than the animal’s BMR – by about two to four times. We humans are more sedentary (less active) than the typical animal, so we have an average daily metabolic rate of only about 1.5 times our BMR.
An animal’s metabolic rate determines how much food it must consume to maintain its body at a constant mass. If an animal doesn’t eat enough food to replace the energy it uses up, it will lose body mass (as glycogen, fats, and other macromolecules are burned for fuel). On the other hand, if an animal eats more food than it needs to replace the energy it uses, there will be leftover chemical energy that is stored by the body as glycogen or fat. This is the basis of weight loss and weight gain in humans as well as other animals.
Scientist Spotlight: Dr. Cara Ocobock
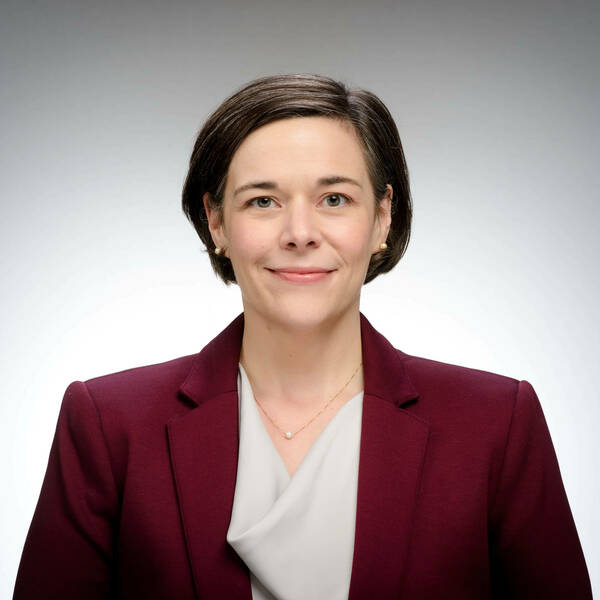
Woman the Hunter – Lunch Break Science featuring Dr. Cara Ocobock
Watch this fascinating interview with Cara Ocobock on Lunch Break Science*. Dr. Ocobock is an associate professor and the director of the Human Energetics Laboratory at Notre Dame University and is an award-winning science communicator. She studies the evolutionary, physiological and behavioral underpinnings of humans in extreme environments (climate) and under extreme conditions (physical activity). In this interview, Dr. Ocobock discusses how her research on women’s physical capabilities and endurance challenges traditional narratives of their role in human evolution.
*Lunch Break Science is a web series featuring short talks and interviews with scientists affiliated with the non-profit Leaky Foundation, which supports scientific research and education on human evolution.
Torpor, hibernation, and estivation
Some animals respond to environmental cues by slowing down their metabolic processes and reducing their body temperature, entering what’s known as torpor. Torpor is a state of decreased activity and metabolism that allows animals to survive unfavorable conditions and/or conserve energy.
Torpor may be used over long periods. For instance, some animals go into hibernation, a state in which they slow their metabolism and maintain a reduced body temperature during the winter. Cues that cause animals to enter hibernation include drops in temperature and the shortening of days. Figure 10 below shows a Norway bat in its winter hibernation.
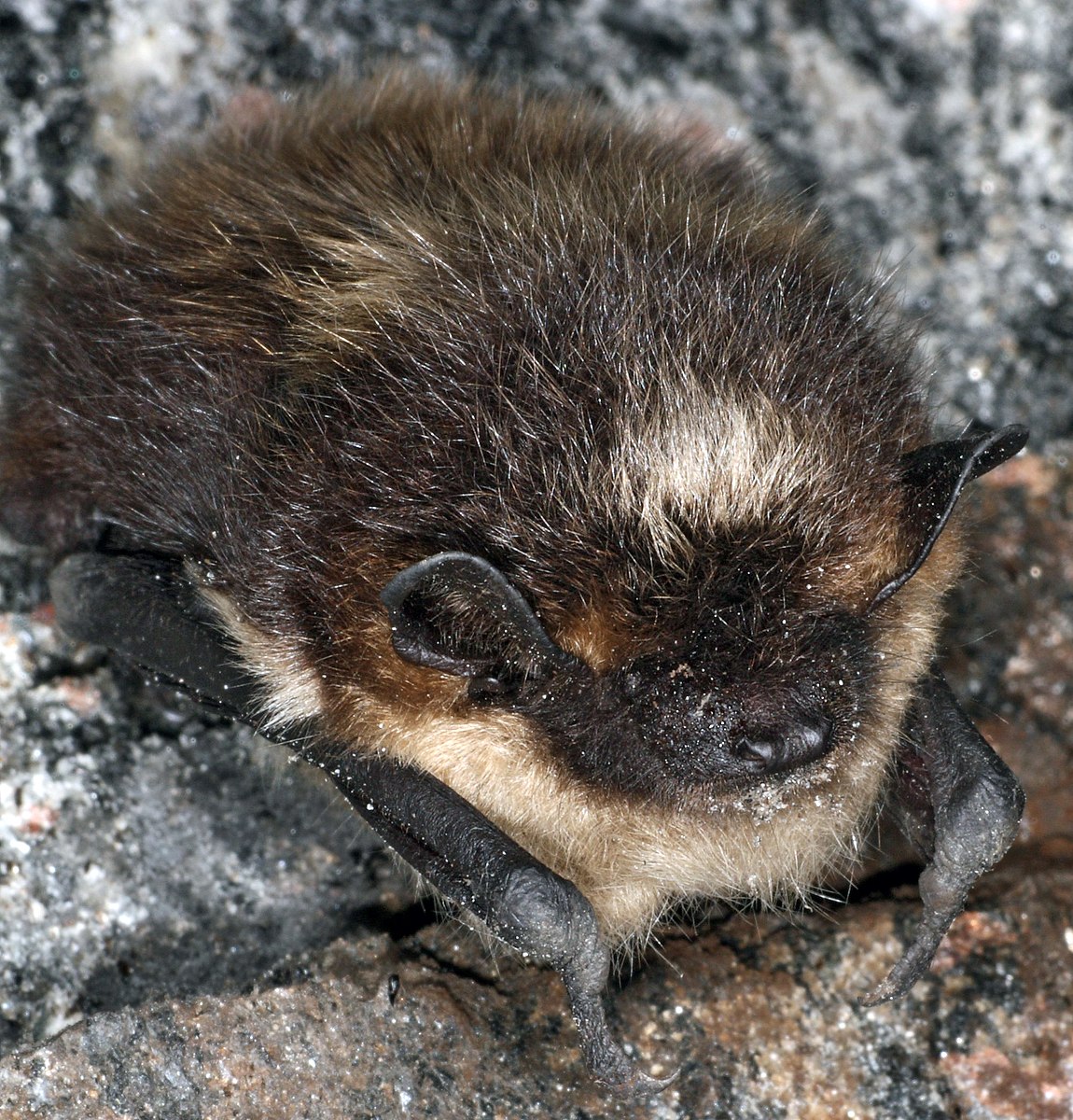
Different animals have different hibernation patterns. For instance, the abdominal temperature of a hibernating ground squirrel may drop as low as 0°C (32°F), but the squirrel must wake up periodically during its hibernation period – possibly to sleep, eat, or do other body maintenance. In contrast, a bear’s internal temperature stays higher, at 31°C (88°F) or above, but the bear can hibernate for its entire winter period without needing to awaken.
The family of alpine marmots in the next video from PBS Nature is preparing for a half-year hibernation underground:
Learn how a Vancouver Island marmot wakes up from a 7-month hibernation in this clip from the BBC wildlife show Animals: The Inside Story:
Some animals enter an extended period of torpor during the summer months, when there are high temperatures and little water. In this case, the extended torpor is called estivation. Some desert animals estivate in response to dry conditions, and this shift helps them survive the harshest months of the year. The snails in Figure 11 below climb to the tops of fence posts to estivate.
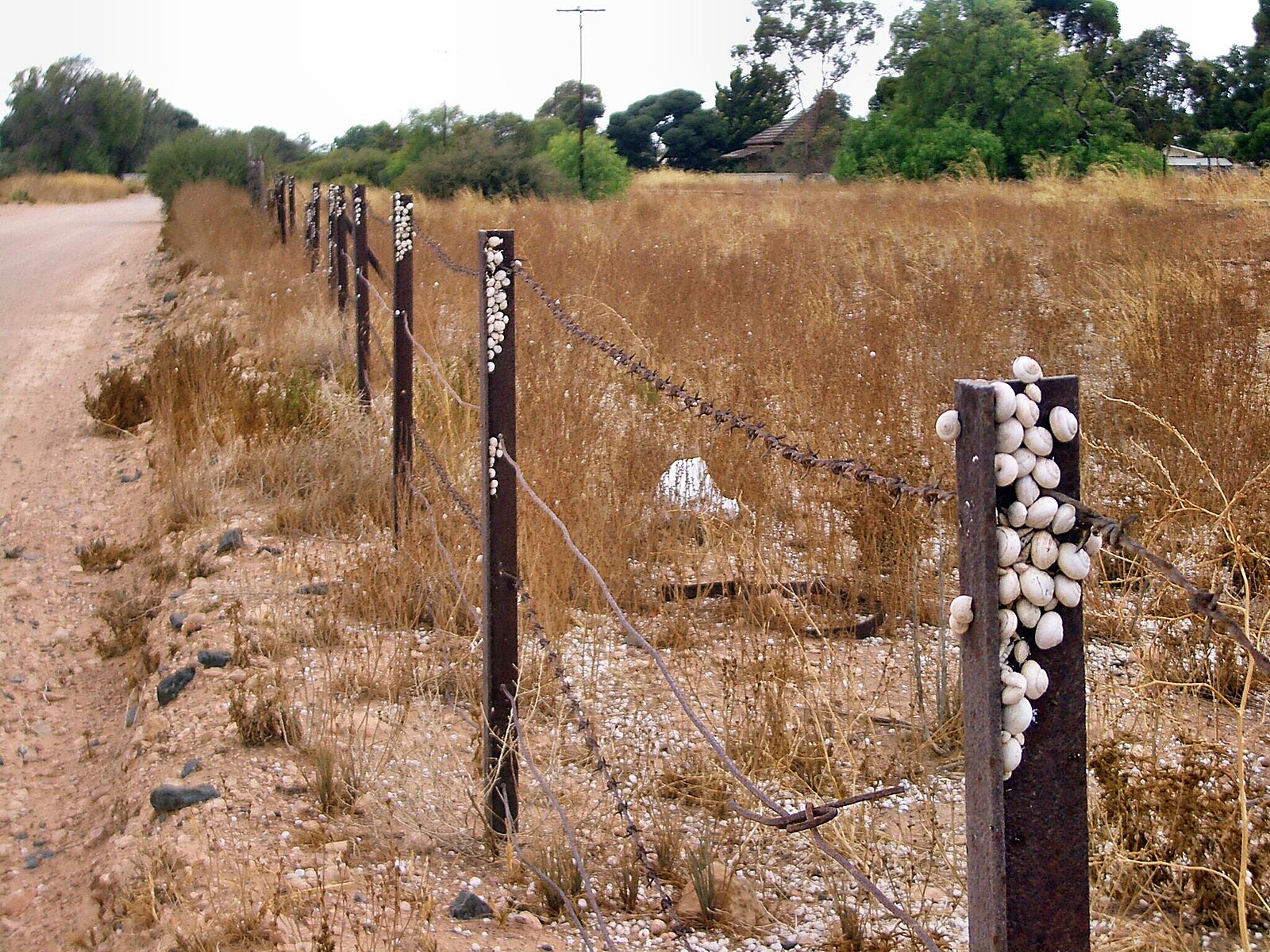
Torpor can also last for short periods. Daily torpor can be sporadic, in response to unfavorable conditions, or can repeat in a predictable pattern. For instance, some small endotherms such as dormice reduce the amount of energy they need (and thus, food they must consume) by entering torpor during the part of the day that is coldest, when they would otherwise need to use a lot of energy to produce metabolic heat and maintain body temperature.
Did I Get It?
Thermoregulation
Why do lizards sunbathe? Why do jackrabbits have huge ears? Why do dogs pant when they’re hot? Animals have quite a few different ways to regulate body temperature! These thermoregulatory strategies let them live in different environments, including some that are pretty extreme.
Polar bears and penguins, for instance, maintain a high body temperature in their chilly homes at the poles, while kangaroo rats, iguanas, and rattlesnakes thrive in Death Valley, where summertime highs are over 100°F (38°C) (Figure 12).
Let’s take a closer look at some behavioral strategies, physiological processes, and anatomical features that help animals regulate body temperature.
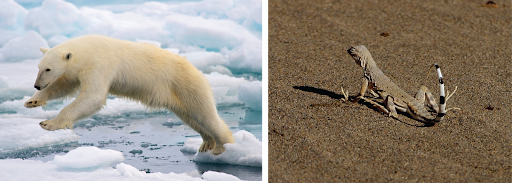
Mechanisms of thermoregulation
Both endotherms and ectotherms have adaptations—features that arose by natural selection—that help them maintain a healthy body temperature. These adaptations can be behavioral, anatomical, or physiological. Some adaptations increase heat production in endotherms when it’s cold. Others, in both endotherms and ectotherms, increase or decrease exchange of heat with the environment.
We will look at three broad categories of thermoregulatory mechanisms in this article:
- Changing behavior
- Increasing metabolic heat production
- Controlling the exchange of heat with the environment
Behavioral strategies
How do you regulate your body temperature using behavior? On a hot day, you might go for a swim, drink some cold water, or sit in the shade. On a cold day, you might put on a coat, sit in a cozy corner, or eat a bowl of hot soup.
Nonhuman animals have similar types of behaviors. For instance, elephants spray themselves with water to cool down on a hot day, and many animals seek shade when they get too warm. On the other hand, lizards often bask on a hot rock to warm up, and penguin chicks huddle in a group to retain heat.
Some ectotherms are so good at using behavioral strategies for temperature regulation that they maintain a fairly stable body temperature, even though they don’t use metabolic heat to do so.
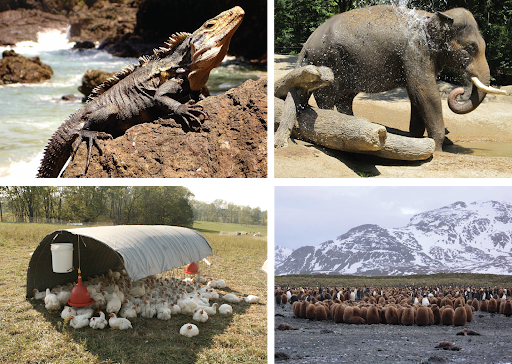
Increasing heat production—thermogenesis
Endotherms have various ways of increasing metabolic heat production, or thermogenesis, in response to cold environments.
One way to produce metabolic heat is through muscle contraction—for example, if you shiver uncontrollably when you’re very cold. Both deliberate movements—such as rubbing your hands together or going for a brisk walk—and shivering increase muscle activity and thus boost heat production.
Nonshivering thermogenesis provides another mechanism for heat production. This mechanism depends on specialized fat tissue known as brown fat, or brown adipose tissue. Some mammals, especially hibernators and baby animals, have lots of brown fat. Brown fat contains many mitochondria with special proteins that let them release energy from fuel molecules directly as heat instead of channeling it into formation of the energy carrier ATP.
Controlling the loss and gain of heat
Animals also have body structures and physiological responses that control how much heat they exchange with the environment:
- Circulatory mechanisms, such as altering blood flow patterns
- Insulation, such as fur, fat, or feathers
- Evaporative mechanisms, such as panting and sweating
Circulatory mechanisms
The body’s surface is the main site for heat exchange with the environment. Controlling the flow of blood to the skin is an important way to control the rate of heat loss to—or gain from—the surroundings.
In endotherms, warm blood from the body’s core typically loses heat to the environment as it passes near the skin. Shrinking the diameter of blood vessels that supply the skin, a process known as vasoconstriction, reduces blood flow and helps retain heat (Figure 14).
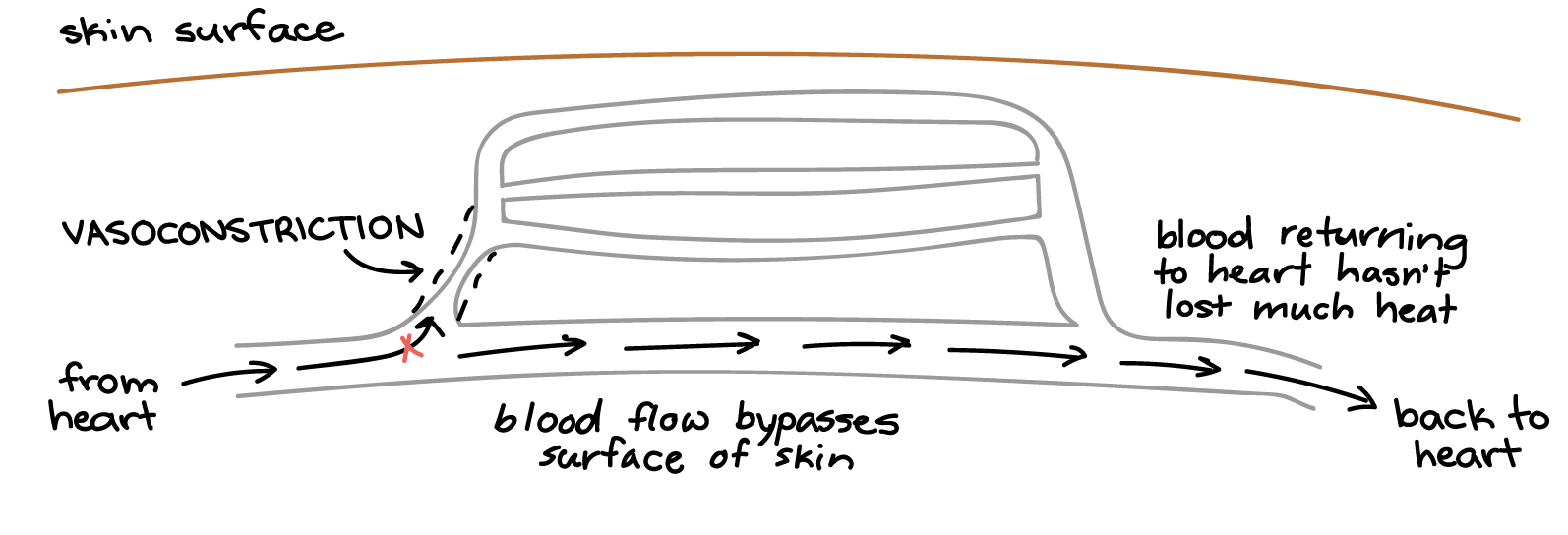
On the other hand, when an endotherm needs to get rid of heat—say, after running hard to escape a predator—these blood vessels get wider, or dilate. This process is called vasodilation. Vasodilation increases blood flow to the skin and helps the animal lose some of its extra heat to the environment (Figure 15).
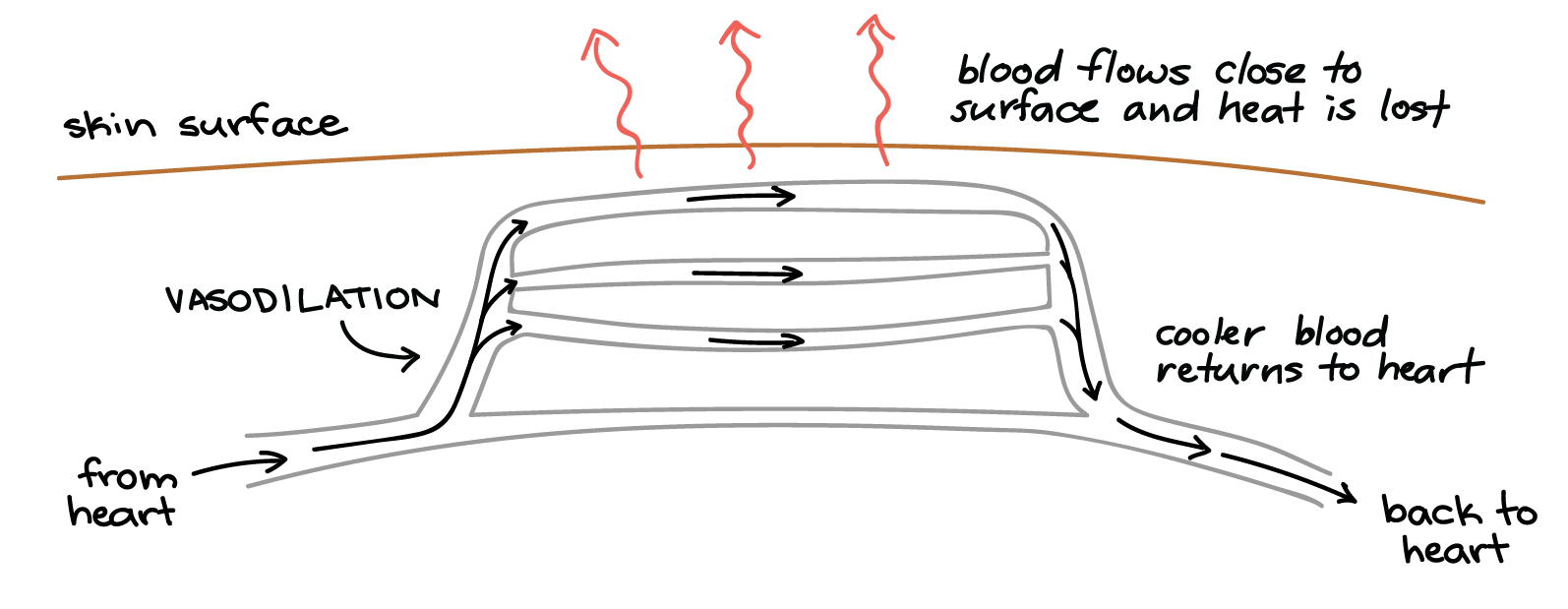
Furry mammals often have special networks of blood vessels for heat exchange located in areas of bare skin. For example, jackrabbits have large ears with an extensive network of blood vessels that allow rapid heat loss. This adaptation helps them live in hot desert environments (Figure 16).
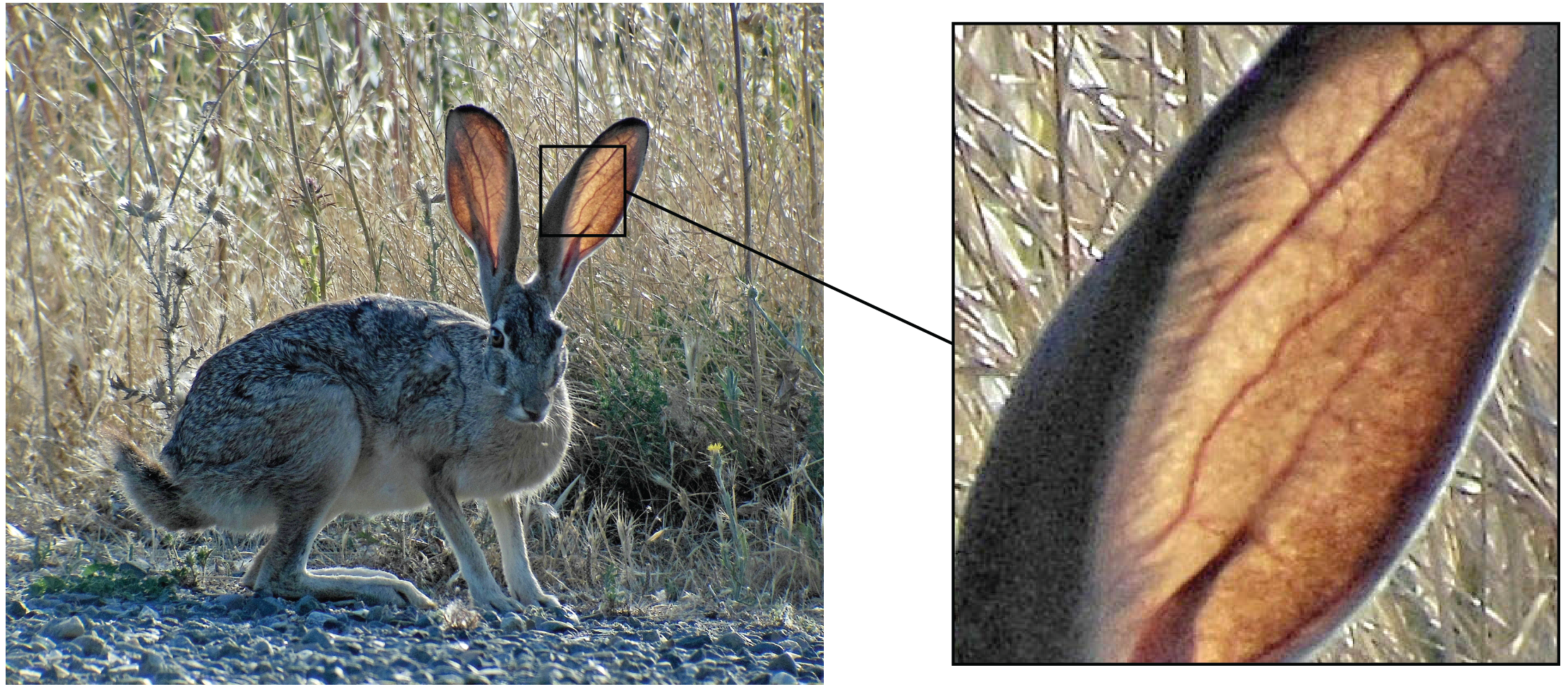
Some ectotherms also regulate blood flow to the skin as a way to conserve heat. For instance, iguanas reduce blood flow to the skin when they go swimming in cold water to help retain the heat they soaked up while on land.
Many birds and mammals have countercurrent heat exchangers, circulatory adaptations that allow heat to be transferred from blood vessels containing warmer blood to those containing cooler blood. To see how this works, let’s look at an example.
In the leg of a wading bird, the artery that runs down the leg carries warm blood from the body. The artery is positioned right alongside a vein that carries cold blood up from the foot. The descending, warm blood passes much of its heat to the ascending, cold blood by conduction (Figure 17). This means that less heat will be lost in the foot due to the reduced temperature difference between the cooled blood and the surroundings and that the blood moving back into the body’s core will be relatively warm, keeping the core from getting cold.
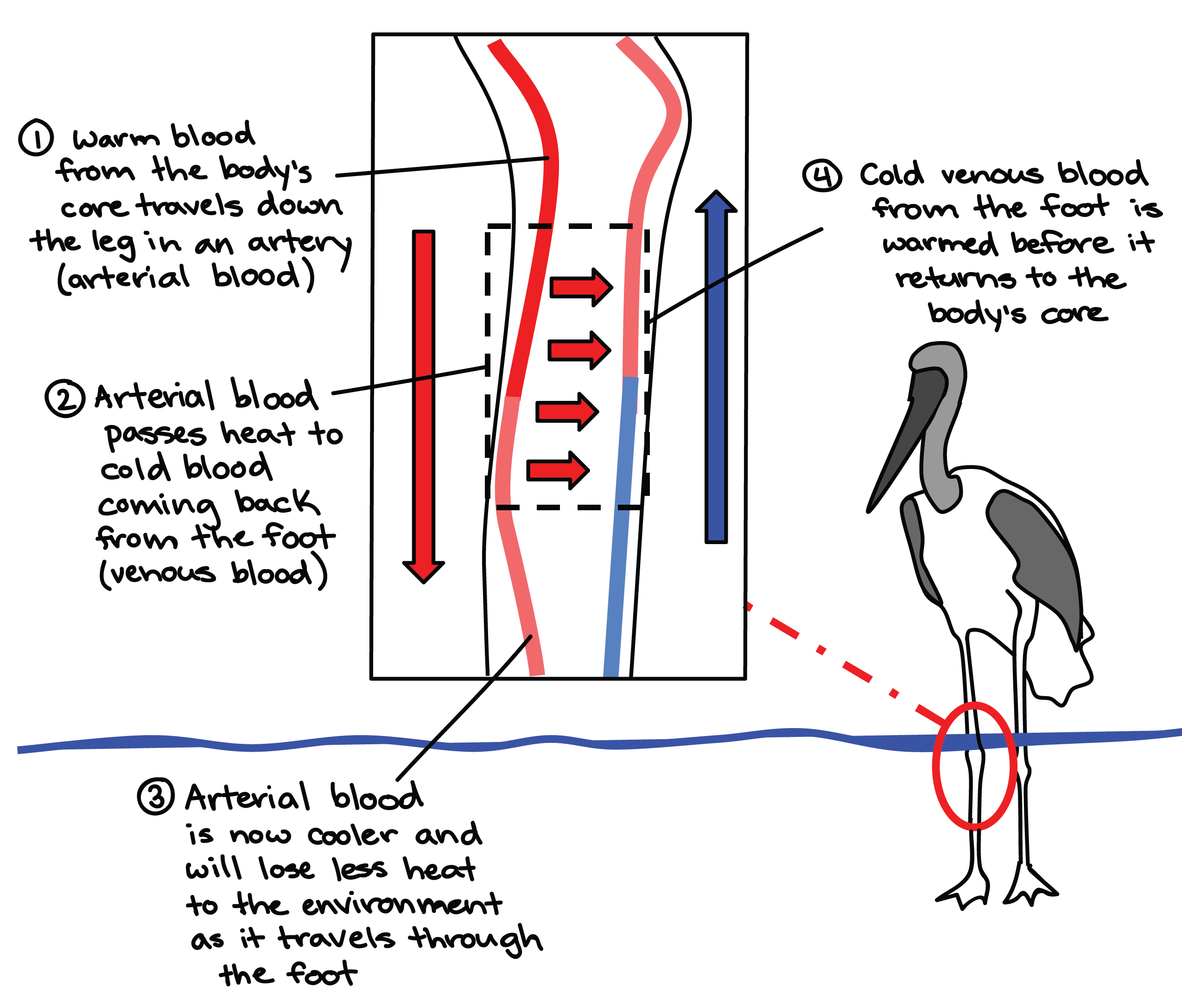
Insulation
Another way to minimize heat loss to the environment is through insulation. Birds use feathers, and most mammals use hair or fur, to trap a layer of air next to the skin and reduce heat transfer to the environment. Marine mammals like whales use blubber, a thick layer of fat, as a heavy-duty form of insulation.
In cold weather, birds fluff their feathers and animals raise their fur to thicken the insulating layer. The same response in people—goosebumps—is not so effective because of our limited body hair (Figure 18). So, most of us wear sweaters!
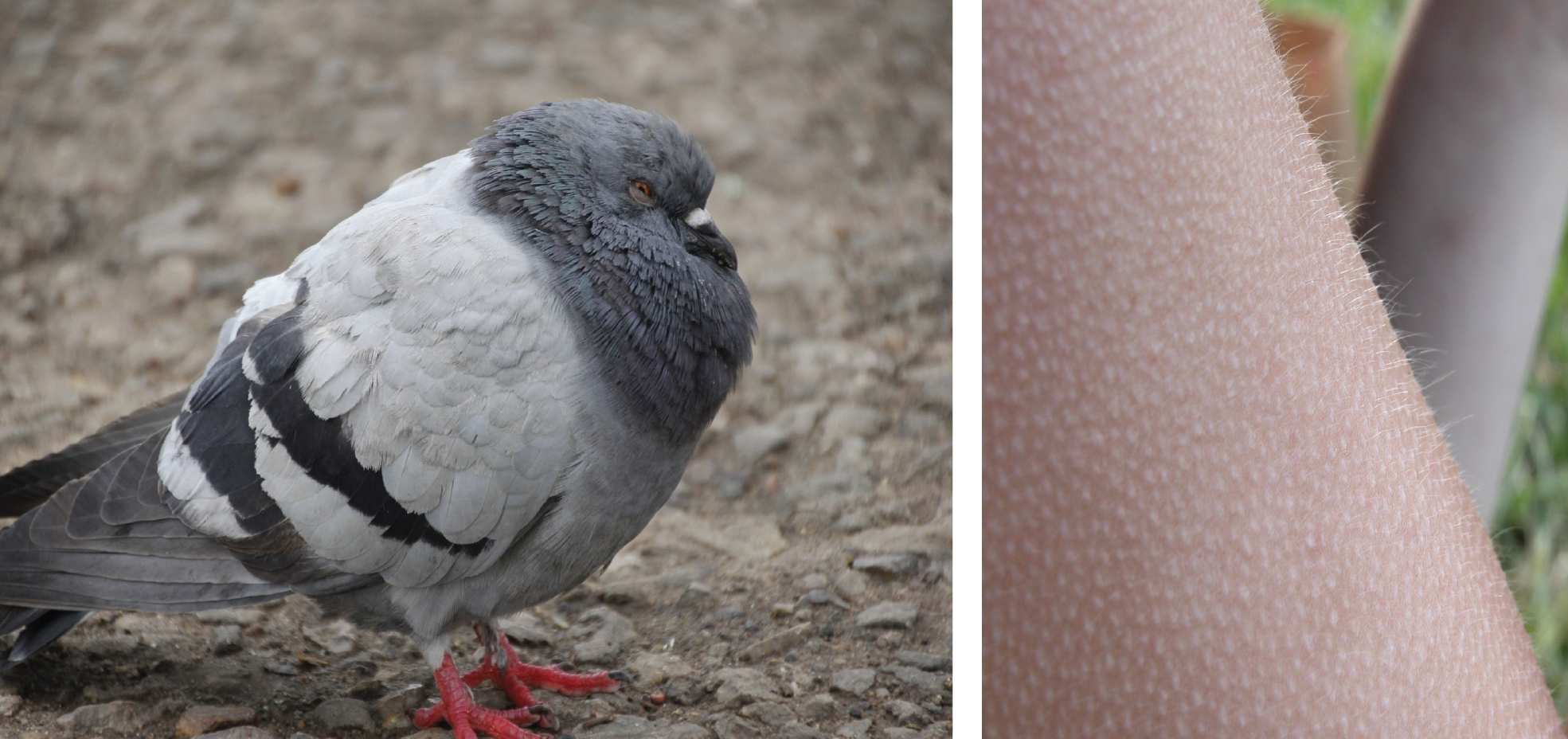
Evaporative mechanisms
Land animals often lose water from their skin, mouth, and nose by evaporation into the air. Evaporation removes heat and can act as a cooling mechanism.
For instance, many mammals can activate mechanisms like sweating and panting to increase evaporative cooling in response to high body temperature (Figure 19).
In sweating, glands in the skin release water containing various ions—the “electrolytes” we replenish with sports drinks. Only mammals sweat. Learn more about why sweating cools you down in this video from Khan Academy with LeBron James. Then, check out the next video from the BBC Planet Earth to learn about a unique evaporative body-cooling behavior in kangaroos!
In panting, an animal breathes rapidly and shallowly with its mouth open to increase evaporation from the surfaces of the mouth. Both mammals and birds pant, or at least use similar breathing strategies to cool down.
In some species, such as dogs, evaporative cooling from panting combined with a countercurrent heat exchanger helps keep the brain from overheating!
Although panting and sweating are effective cooling mechanisms, these active processes have the unwanted side effect of increasing the metabolic rate and thereby heat production. In addition, panting and sweating cause the animal to lose water and can result in dehydration—always make sure your dog has lots of water available on a hot day! Sweating also depletes the body of electrolytes, which must be replaced to avoid an imbalance.
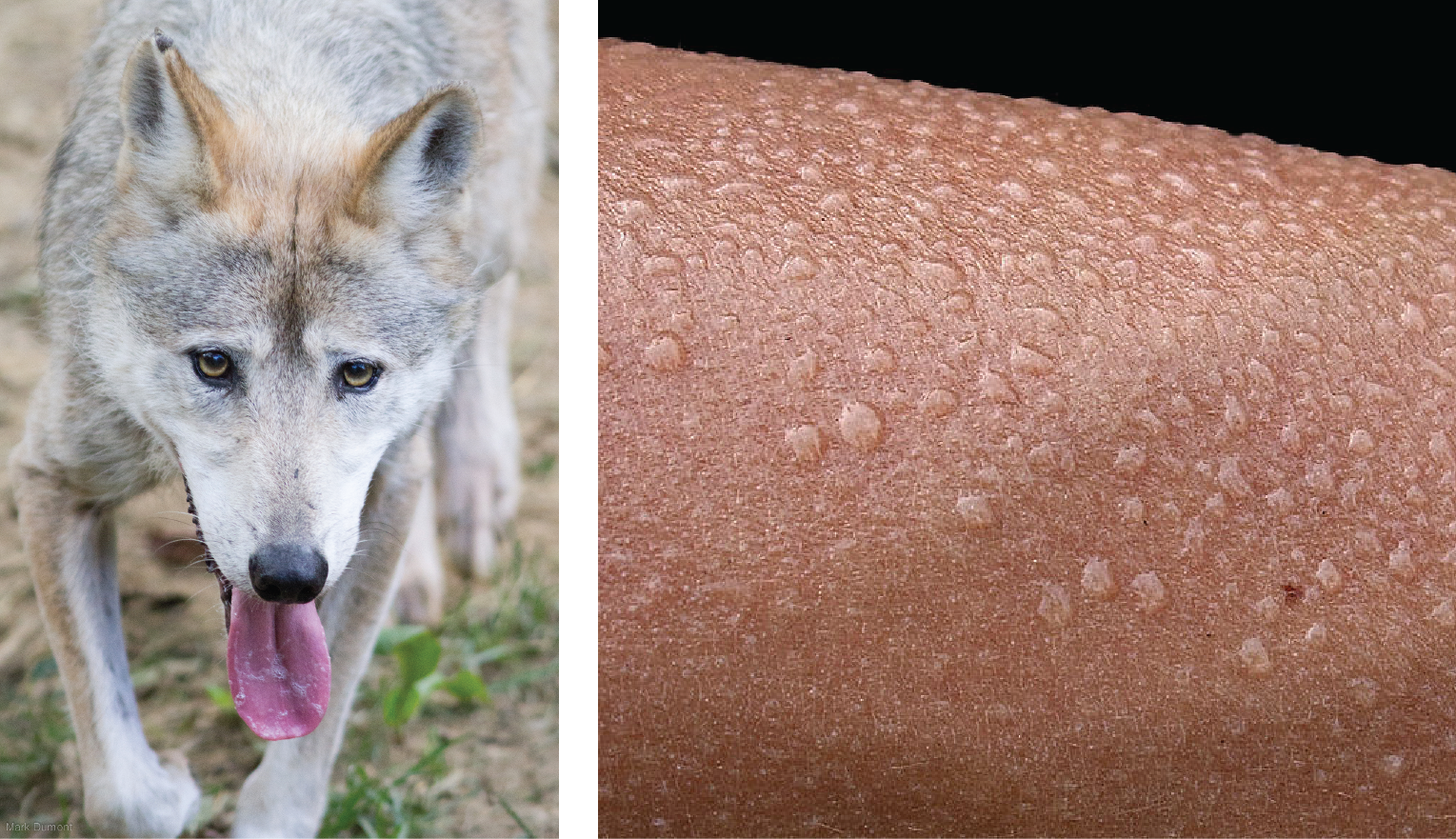
CC Licensed Content, Shared Previously, Included in An Introduction to Animal Physiology
- Biology 2e. Authors: Mary Ann Clark, Matthew Douglas and Jung Choi. Provided by: OpenStax CNX. Located at: Biology 2e. License: CC BY: Attribution 4.0.
- Biology for Majors II. Authors: Shelly Carter and Monisha Scott. Provided by: Lumen Learning. Located at: Biology for Majors II | Simple Book Production. License: CC BY: Attribution 4.0.
- Endotherms & ectotherms. Provided by: Khan Academy. Located at: Endotherms & ectotherms (article) | Ecology | Khan Academy License: CC BY-NC-SA 4.0
- Metabolic rate. Provided by: Khan Academy. Located at: Metabolic rate (article) | Ecology | Khan Academy. License: CC BY-NC-SA 4.0
- Thermoregulation. Provided by: Khan Academy. Located at: Thermoregulation | Temperature regulation strategies (article) | Ecology | Khan Academy. License: CC BY-NC-SA 4.0