Evidence for Evolution
Section Goals
By the end of this section, you will be able to do the following:
- Outline physical evidence that supports the theory of evolution
- Outline biological evidence that supports the theory of evolution
- Refute common misconceptions about evolution
The evidence for evolution is compelling and extensive. Looking at every level of organization in living systems, biologists see the signature of past and present evolution. Darwin dedicated a large portion of his book, On the Origin of Species, to identifying patterns in nature that were consistent with evolution, and since Darwin, our understanding has become clearer and broader.
Physical Evidence
Fossils
Fossils provide solid evidence that organisms from the past are not the same as those found today, and fossils show a progression of evolution. Scientists determine the age of fossils and categorize them from all over the world to determine when the organisms lived relative to each other. The resulting fossil record tells the story of the past and shows the evolution of form over millions of years (Figure 1a). For example, scientists have recovered highly detailed records showing the evolution of humans and horses (Figure 1b).
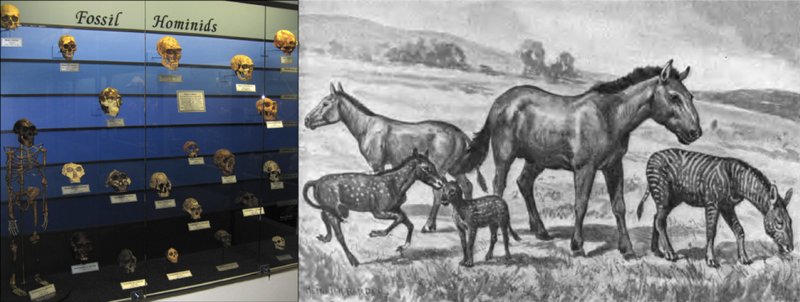
Anatomy and Embryology
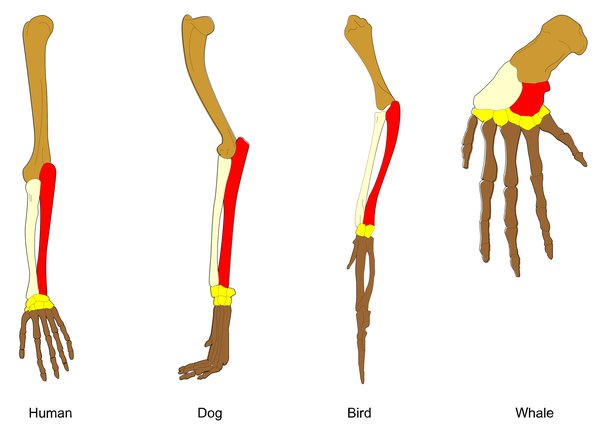
Another type of evidence for evolution is the presence of structures in organisms that share the same basic form. For example, the bones in the appendages of a human, dog, bird, and whale all share the same overall construction (Figure 2) resulting from their origin in the appendages of a common ancestor. Over time, evolution led to changes in the shapes and sizes of these bones in different species, but they have maintained the same overall layout. Scientists call these synonymous parts homologous structures.
Some structures exist in organisms that have no apparent function at all, and appear to be residual parts from a past common ancestor. These unused structures without function are called vestigial structures. Some examples of vestigial structures are wings on flightless birds, leaves on some cacti, and hind leg bones in whales.
Another evidence of evolution is the convergence of form in organisms that share similar environments. For example, species of unrelated animals, such as the arctic fox and ptarmigan, living in the arctic region have been selected for seasonal white phenotypes during winter to blend with the snow and ice (Figure 3). These similarities occur not because of common ancestry, but because of similar selection pressures—the benefits of not being seen by predators.
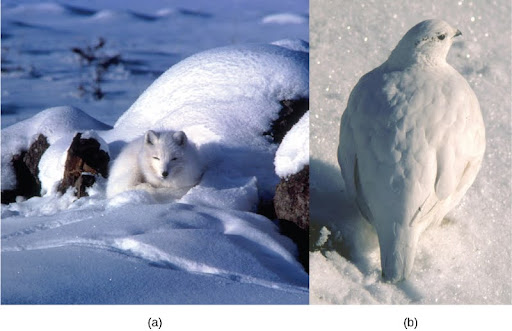
Embryology, the study of the development of the anatomy of an organism to its adult form, also provides evidence of relatedness between now widely divergent groups of organisms. Mutational tweaking in the embryo can have such magnified consequences in the adult that embryo formation tends to be conserved. As a result, structures that are absent in some groups often appear in their embryonic forms and disappear by the time the adult or juvenile form is reached. For example, all vertebrate embryos, including humans, exhibit gill slits and tails at some point in their early development. These disappear in the adults of terrestrial groups but are maintained in adult forms of aquatic groups such as fish and some amphibians. Great ape embryos, including humans, have a tail structure during their development that is lost by the time of birth.
Misleading Appearances
Some organisms may be very closely related, even though a minor genetic change caused a major morphological difference to make them look quite different. Similarly, unrelated organisms may be distantly related, but appear very much alike. This usually happens because both organisms in common adaptations that evolved within similar environmental conditions, like the white phenotypes in the Arctic fox and ptarmigan in Figure 3 above. When similar characteristics occur because of environmental constraints and not due to a close evolutionary relationship, it is an analogy or homoplasy. For example, insects use wings to fly like bats and birds, but the wing structure and embryonic origin are completely different. These are analogous structures (Figure 4). Analogous structures are the result of convergent evolution.
Similar traits can be either homologous or analogous. Homologous structures share a similar embryonic origin. Analogous organs have a similar function. For example, the bones in a whale’s front flipper are homologous to the bones in the human arm. These structures are not analogous. A butterfly and a bird’s wings are analogous, not homologous. Some structures are both analogous and homologous: bird and bat wings are both homologous and analogous. Bird and bat wings are analogous to wings. As forelimbs, they are homologous. Birds and bats did not inherit wings from a common ancestor with wings, but they did inherit forelimbs from a common ancestor with forelimbs. Scientists must determine which type of similarity a feature exhibits to decipher the organisms’ phylogeny.
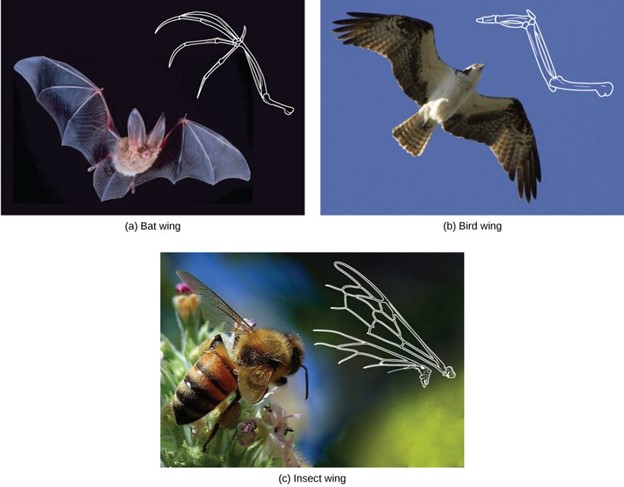
Click through this interactive module to learn more about homologies and convergent traits, and to test your understanding!
Biological Evidence
Biogeography
The geographic distribution of organisms on the planet follows patterns that are best explained by evolution in conjunction with the movement of tectonic plates over geological time. Broad groups that evolved before the breakup of the supercontinent Pangaea (about 200 million years ago) are distributed worldwide. Groups that evolved since the breakup appear uniquely in regions of the planet, such as the unique flora and fauna of northern continents that formed from the supercontinent Laurasia and of the southern continents that formed from the supercontinent Gondwana. The presence of members of the plant family Proteaceae in Australia, southern Africa, and South America is best due to their appearance prior to the southern supercontinent Gondwana breaking up.
The great diversification of marsupials in Australia and the absence of other mammals reflect Australia’s long isolation. Australia has an abundance of endemic species—species found nowhere else—which is typical of islands whose isolation by expanses of water prevents species migration. Over time, these species diverge evolutionarily into new species that look very different from their ancestors that may exist on the mainland. The marsupials of Australia, the finches on the Galápagos, and many species on the Hawaiian Islands are all unique to their one point of origin, yet they display distant relationships to ancestral species on the mainland.
Molecular Evidence
DNA can provide a lot of information to help us study relationships among organisms. We can look at genes from multiple taxa to determine if they are similar or different to help us understand how closely or distantly related they are to one another. Homeotic genes are sections of DNA that play a key role in the developmental process as embryos undergo all sorts of changes. Homeotic genes act as on-and-off switches to help with the positioning of cells within the organism’s body plan.
One group of homeotic genes that turn out to be very helpful for understanding evolution is hox genes. Hox genes determine the form, number, and evolution of repeating parts, such as the number and type of vertebrae in animals. The role of hox genes is to specify positional identity in the embryo, which will lead to corresponding structures in the adult. For example, at least eight different Hox genes are critical for the development of different body segments in fruit flies (Figure 5). Hox genes also affect the orientation of segments such that the anterior-to-posterior orientation is maintained. Scientists have learned that hox genes are part of a “toolbox” of genes that can be shuffled and rearranged to create the diversity of species we find on the planet.
When studying animal species, we find animal species with more simple body structures do not have as many hox genes as those species with more complex bodies. For example, a mammal such as a mouse will have many more hox genes than a flatworm. Humans have over 200 homeotic genes, and out of that, 39 are hox genes.
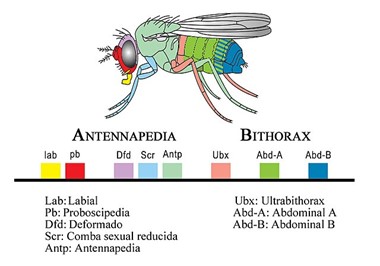
When looking more closely at homeotic genes, scientists discovered a 180-base-pair sequence to be identical in a wide array of animal species. This 180 base-pair sequence is called the homeobox and is highly conserved across evolutionary time (and it was first discovered in fruit flies). This suggests that hox genes arose very early in evolutionary time. The shared homeobox sequence suggests an ancestral gene was present and duplicated multiple times over evolutionary time to give rise to what we find today. Scientists get very excited about the homeobox because mutations in this gene can cause dramatic developmental changes in body parts and can lead to speciation.
Hox genes, as mentioned above, are conserved across species. We find some of the human hox genes are homologous to those in the fruit fly. In experiments with mice, the Hox10 genes turn the “rib” genes off which are normally active in the lower back, but not needed. When scientists experimentally manipulate these genes, they can cause ribs to grow in the vertebrae of the lower back. As such, we can see hox genes dictating arms versus legs as well as differentiating specific fingers, like a thumb or a pinky. The variation on the theme of the hox genes will give an organism a variety of traits and can lead to speciation.
Click through this animation to see how these master genes control basic fruit fly body plans. Visit this resource page to further explore how hox genes hold clues about evolutionary relationships!
DNA sequences have also shed light on some of the mechanisms of evolution. For example, it is clear that the evolution of new functions for proteins commonly occurs after gene duplication events that allow the free modification of one copy by mutation, selection, or drift (changes in a population’s gene pool resulting from chance), while the other copy continues to produce a functional protein.
Check out the next video to review several varieties of evidence that support the Theory of Evolution:
Did I Get It?
Misconceptions about Evolution
Although the theory of evolution generated some controversy when it was first proposed, it was almost universally accepted by biologists, particularly younger biologists, within 20 years after the publication of On the Origin of Species. Nevertheless, the theory of evolution is a difficult concept, and misconceptions or misrepresentations about how it works are not uncommon. Here are some of the big ones:
Evolution Is Just a Theory
Critics of the theory of evolution dismiss its importance by purposefully confounding the everyday usage of the word “theory” with the way scientists use the word. In science, a “theory” is understood to be a body of thoroughly tested and verified explanations for a set of observations of the natural world. Scientists have a theory of the atom, a theory of gravity, and the theory of relativity, each of which describes understood facts about the world. In the same way, the theory of evolution describes facts about the living world. As such, a theory in science has survived significant efforts to discredit it by scientists.
In contrast, a “theory” in the common vernacular is a word meaning a guess or suggested explanation; this meaning is more akin to the scientific concept of “hypothesis.” When critics of evolution say evolution is “just a theory,” they are implying that there is little evidence supporting it and that it is still being rigorously tested. This use of the word theory is a mischaracterization.
Individuals Evolve
Evolution is the change in the genetic composition of a population over time, specifically over generations, resulting from the differential reproduction of individuals with certain alleles. Individuals do change over their lifetime, obviously, but this is called development and involves changes programmed by the set of genes the individual acquired at birth in coordination with the individual’s environment. When thinking about the evolution of a characteristic, it is probably best to think about it as the change of the average value of the characteristic in the population over time. For example, when natural selection leads to bill-size changes in medium-ground finches in the Galápagos, this does not mean that individual bills on the finches are changing. If one measures the average bill size among all individuals in the population at one time and then measures the average bill size in the population several years later, this average value will be different as a result of evolution. Although some individuals may survive from the first time to the second, they will still have the same bill size; however, there will be many new individuals that contribute to the shift in average bill size.
Organisms Evolve on Purpose
Statements such as “organisms evolve in response to a change in an environment” are quite common, but such statements can lead to two types of misunderstandings. First, the statement must not be understood to mean that individual organisms evolve. The statement is shorthand for “a population evolves in response to a changing environment.” However, a second misunderstanding may arise by interpreting the statement to mean that the evolution is somehow intentional. A changed environment results in some individuals in the population, those with particular phenotypes, benefiting and therefore producing proportionately more offspring than other phenotypes. This results in a change in the population if the characteristics are genetically determined.
It is also important to understand that the variation that natural selection works on is already in a population and does not arise in response to an environmental change. For example, applying antibiotics to a population of bacteria will, over time, select a population of bacteria that are resistant to antibiotics. The resistance, which is caused by a gene, did not arise by mutation because of the application of the antibiotic. The gene for resistance was already present in the gene pool of the bacteria, likely at a low frequency. The antibiotic, which kills the bacterial cells without the resistance gene, strongly selects resistant individuals, since these would be the only ones that survive and divide. Experiments have demonstrated that mutations for antibiotic resistance do not arise as a result of antibiotics.
In a larger sense, evolution is not goal-directed. Species do not become “better” over time; they simply track their changing environment with adaptations that maximize their reproduction in a particular environment at a particular time. Evolution has no goal of making faster, bigger, more complex, or even smarter species, despite the commonness of this kind of language in popular discourse. What characteristics evolve in a species are a function of the variation present and the environment, both of which are constantly changing in a non-directional way. What trait is fit in one environment at one time may well be fatal at some point in the future. This property of traits holds equally well for a species of insect as it does for the human species.
Evolution Explains the Origin of Life
It is a common misunderstanding that evolution includes an explanation of life’s origins. Conversely, some of the theory’s critics believe that it cannot explain the origin of life. The theory does not try to explain the origin of life. The theory of evolution explains how populations change over time and how life diversifies the origin of species. It does not shed light on the beginnings of life, including the origins of the first cells, which is how life is defined. The mechanisms of the origin of life on Earth are a particularly difficult problem because it occurred a very long time ago, and presumably it just occurred once. Importantly, biologists believe that the presence of life on Earth precludes the possibility that the events that led to life on Earth can be repeated because the intermediate stages would immediately become food for existing living things.
However, once a mechanism of inheritance was in place in the form of a molecule like DNA either within a cell or pre-cell, these entities would be subject to the principle of natural selection. More effective reproducers would increase in frequency at the expense of inefficient reproducers. So while evolution does not explain the origin of life, it may have something to say about some of the processes operating once pre-living entities acquired certain properties.
Check out this more comprehensive list of common misconceptions about evolution from the Understanding Evolution site.
Did I Get It?
Since Darwin developed his ideas on descent with modification and the pressures of natural selection, a variety of evidence has been gathered supporting the theory of evolution. Fossil evidence shows the changes in lineages over millions of years, such as in hominids and horses. Studying anatomy allows scientists to identify homologous structures across diverse groups of related organisms, such as leg bones. Vestigial structures also offer clues to common ancestors. Using embryology, scientists can identify common ancestors through structures present only during development and not in the adult form. Biogeography offers further clues about evolutionary relationships. The presence of related organisms across continents indicates when these organisms may have evolved. For example, some flora and fauna of the northern continents are similar across these landmasses but distinct from those of the southern continents. Islands such as Australia and the Galapagos chain often have unique species that evolved after these landmasses separated from the mainland. Finally, molecular biology provides data supporting the theory of evolution. In particular, the universality of DNA and near universality of the genetic code for proteins shows that all life once shared a common ancestor. DNA also provides clues into how evolution may have happened. Gene duplications allow one copy to undergo mutational events without harming an organism, as one copy continues to produce functional protein.
Many misconceptions exist about the theory of evolution—including some perpetuated by critics of the theory. First, evolution as a scientific theory means that it has years of observation and accumulated data supporting it. It is not “just a theory” as a person may say in common vernacular.
Another misconception is that individuals evolve, though in fact it is populations that evolve over time. Individuals simply carry mutations. Furthermore, these mutations neither arise on purpose nor do they arise in response to environmental pressure. Instead, mutations in DNA happen spontaneously and are already present in individuals of a population when selective pressure occurs. Once the environment begins to favor a particular trait, then those individuals already carrying that mutation will have a selective advantage and are likely to survive better and outproduce others without adaptation.
Finally, the theory of evolution does not address the origins of life on this planet. Scientists believe that we cannot repeat the circumstances that led to life on Earth because, at this time, life already exists. The presence of life has so dramatically changed the environment that the origins cannot be totally produced for study.
CC Licensed Content, Shared Previously, Included in Mechanisms of Evolution
- Biology 2e. Authors: Mary Ann Clark, Matthew Douglas, and Jung Choi. Provided by: OpenStax CNX. Located at: Biology 2e. License: CC BY: Attribution 4.0.
- Biology for Majors II. Authors: Shelly Carter and Monisha Scott. Provided by: Lumen Learning. Located at: Biology for Majors II | Simple Book Production. License: CC BY: Attribution 4.0.
- An Interactive Introduction to Organismal and Molecular Biology, 2nd ed. Author: Andrea Bierema. Provided by: Pressbooks. Located at: An Interactive Introduction to Organismal and Molecular Biology, 2nd ed. – Simple Book Publishing. License: CC BY-NC-SA 4.0
- Introductory Biology: Ecology, Evolution and Biodiversity. Author: Erica Kosal. Provided by: Pressbooks. Located at: Introductory Biology: Ecology, Evolution, and Biodiversity – Simple Book Publishing. License: CC BY-NC 4.0