Phylogenetic Trees
Section Goals
By the end of this section, you will be able to do the following:
- Discuss the structure and purpose of a phylogenetic tree
- Identify the types of evidence used to construct phylogenetic trees
- Recognize the practical applications phylogenetic trees
This bee and Echinacea flower (Figure 1) could not look more different, yet they are related, as are all living organisms on Earth. By following pathways of similarities and changes—both visible and genetic—scientists seek to map the evolutionary past of how life developed from single-celled organisms to the tremendous collection of creatures that have germinated, crawled, floated, swum, flown, and walked on this planet.
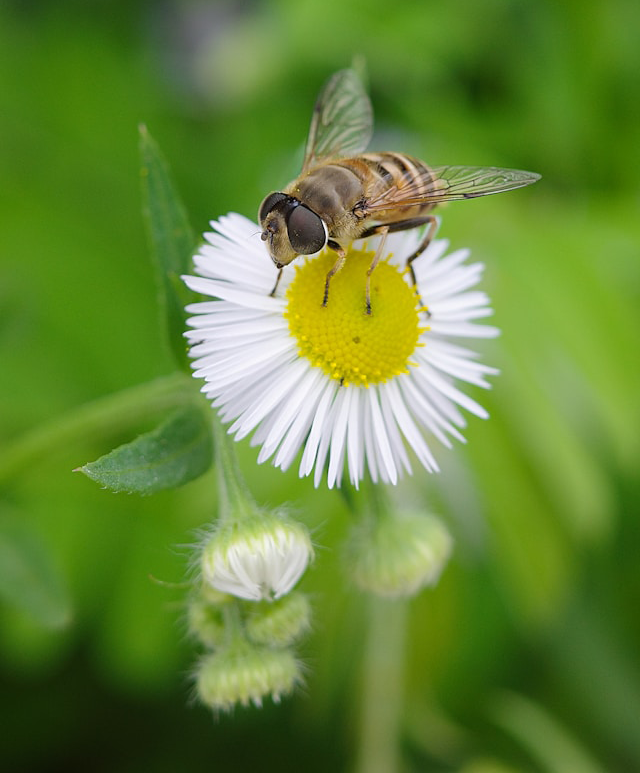
In scientific terms, phylogeny is the evolutionary history and relationship of an organism or group of organisms. A phylogeny describes the organism’s relationships, such as from which organisms it may have evolved or to which species it is most closely related. Phylogenetic relationships provide information on shared ancestry but not necessarily on how organisms are similar or different.
Scientists use a tool called a phylogenetic tree to show the evolutionary pathways and connections among organisms. A phylogenetic tree is a diagram used to reflect evolutionary relationships among organisms or groups of organisms. Scientists consider phylogenetic trees to be a hypothesis of the evolutionary past since one cannot go back to confirm the proposed relationships. In other words, we can construct a “tree of life” to illustrate when different organisms evolved and to show the relationships among different organisms (Figure 2).
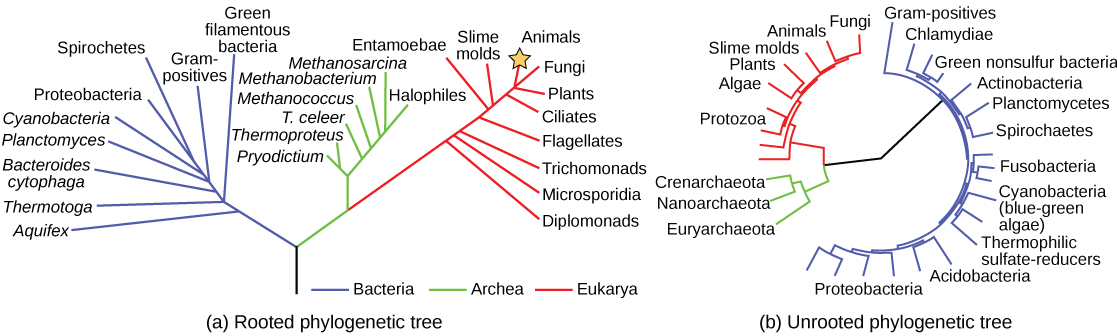
Unlike a taxonomic classification diagram, we can read a phylogenetic tree like a map of evolutionary history. Many phylogenetic trees have a single lineage at the base representing a common ancestor. Scientists call such trees rooted, which means there is a single ancestral lineage (typically drawn from the bottom or left) to which all organisms represented in the diagram relate. Notice in the rooted phylogenetic tree in Figure 2 that the three domains— Bacteria, Archaea, and Eukarya—diverge from a single point and branch off. The small branch that plants and animals (including humans) occupy in this diagram shows how recent and miniscule these groups are compared with other organisms. Unrooted trees do not show a common ancestor but do show relationships among species.
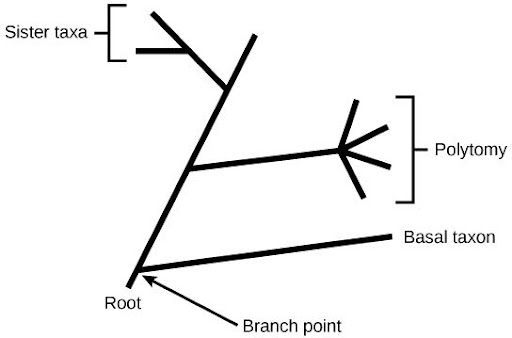
In a rooted tree, the branching indicates evolutionary relationships (Figure 3). The point where a split occurs, called a node, represents where a single lineage evolved into a distinct new one. A lineage that evolved early from the root and remains unbranched is called a basal taxon. When two lineages stem from the same node, they are called sister taxa. A branch with more than two lineages is called a polytomy and serves to illustrate where scientists have not definitively determined all of the relationships. It is important to note that although sister taxa and polytomy do share an ancestor, it does not mean that the groups of organisms split or evolved from each other. Organisms in two taxa may have split apart at a specific branch point, but neither taxon gave rise to the other.
The diagrams above can serve as a pathway to understanding evolutionary history. We can trace the pathway from the origin of life to any individual species by navigating through the evolutionary branches between the two points. Also, by starting with a single species and tracing back towards the “trunk” of the tree, one can discover species’ ancestors, as well as where lineages share a common ancestry. In addition, we can use the tree to study entire groups of organisms.
Another point to mention on phylogenetic tree structure is that rotation at branch points does not change the information. For example, if a branch point rotated and the taxon order changed, this would not alter the information because each taxon’s evolution from the branch point was independent of the other.
Many disciplines within the study of biology contribute to understanding how past and present life evolved over time. These disciplines together contribute to building, updating, and maintaining the “tree of life.” Systematics is the field that scientists use to organize and classify organisms based on evolutionary relationships. Researchers may use data from fossils, from studying the body part structures, or molecules that an organism uses, and DNA analysis. By combining data from many sources, scientists can construct an organism’s phylogeny. Since phylogenetic trees are hypotheses, they will continue to change as researchers discover new types of life and learn new information.
Limitations of Phylogenetic Trees
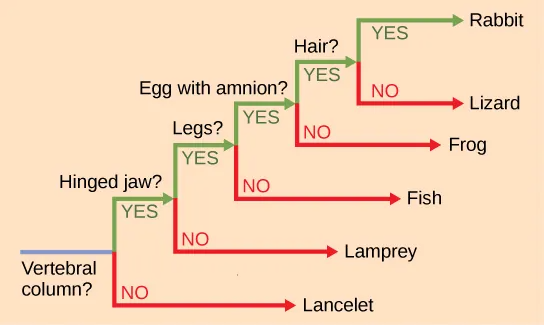
It may be easy to assume that more closely related organisms look more alike, and while this is often the case, it is not always true. If two closely related lineages evolved under significantly varied surroundings, the two groups can appear more different from other groups that are not as closely related. For example, the phylogenetic tree in Figure 4 shows that lizards and rabbits both have amniotic eggs, whereas frogs do not. Yet lizards and frogs appear more similar than lizards and rabbits.
Another aspect of phylogenetic trees is that unless otherwise indicated, the branches may not account for length of time, only the evolutionary order. In other words, a branch’s length does not mean more time passed, nor does a short branch mean less time passed— unless specified on the diagram. For example, in Figure 4, the tree does not indicate how much time passed between the evolution of amniotic eggs and hair. What the tree does show is the order in which things took place. Again using Figure 4, the tree shows that the oldest trait is the vertebral column, followed by hinged jaws, and so forth. Remember that any phylogenetic tree is a part of the greater whole, and like a real tree, it does not grow in only one direction after a new branch develops. Thus, for the organisms in Figure 4, just because a vertebral column evolved does not mean that invertebrate evolution ceased. It only means that a new branch formed. Also, groups that are not closely related but evolve under similar conditions may appear more phenotypically similar to each other than to a close relative.
Head to this website to see interactive exercises that allow you to explore the evolutionary relationships among species.
There are many reasons why understanding phylogeny is important to everyday life in human society. In addition to enhancing our understanding of the evolutionary history of species, our own included, phylogenetic analysis has numerous practical applications.
For botanists, phylogeny acts as a guide to discovering new plants that can be used to benefit people. Think of all the ways humans use plants—food, medicine, and clothing are a few examples. If a plant contains a compound that is effective in treating cancer, scientists might want to examine all of the compounds for other useful drugs.
A research team in China identified a DNA segment that they thought to be common to some medicinal plants in the family Fabaceae (the legume family) (Figure 5). They worked to identify which species had this segment. After testing plant species in this family, the team found a DNA marker (a known location on a chromosome that enabled them to identify the species) present. Then, using the DNA to uncover phylogenetic relationships, the team could identify whether a newly discovered plant was in this family and assess its potential medicinal properties.
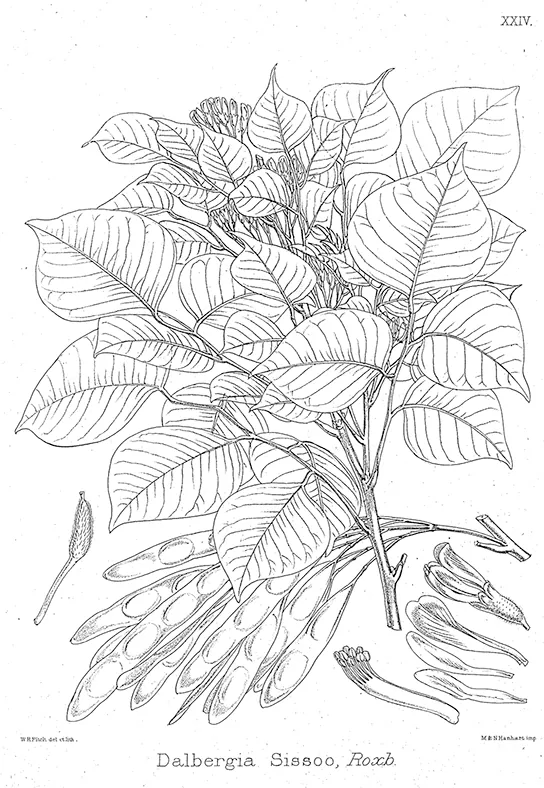
Phylogenetics also helps us understand the evolution and transmission of disease. A 2010 study[1] of MRSA (methicillin-resistant Staphylococcus aureus), an antibiotic-resistant pathogenic bacterium, traced the origin and spread of the strain throughout the past 40 years. The study uncovered the timing and patterns in which the resistant strain moved from its point of origin in Europe to centers of infection and evolution in South America, Asia, North America, and Australasia. The study suggested that introductions of the bacteria to new populations occurred very few times, perhaps only once, and then spread from that limited number of individuals. This is in contrast to the possibility that many individuals carried the bacteria from one place to another. This result suggests that public health officials should concentrate on quickly identifying the contacts of individuals infected with a new strain of bacteria to control its spread.
Yet another area of usefulness for phylogenetic analysis is in conservation. Biologists have argued that it is important to protect species throughout a phylogenetic tree rather than just those from one branch of the tree. Doing this will preserve more of the variation produced by evolution. For example, conservation efforts should focus on a single species without sister species rather than another species that has a cluster of close sister species that recently evolved. If the single evolutionarily distinct species goes extinct, a disproportionate amount of variation from the tree will be lost compared to one species in the cluster of closely related species. A study published in 2007[2] made recommendations for the conservation of mammal species worldwide based on how evolutionarily distinct and at risk of extinction they are. The study found that their recommendations differed from priorities based on simply the level of extinction threat to the species. The study recommended protecting some threatened and valued large mammals such as the orangutans, the giant and lesser pandas, and the African and Asian elephants. But they also found that some much lesser-known species should be protected based on how evolutionary distinct they are. These include a number of rodents, bats, shrews and hedgehogs. In addition, there are some critically endangered species that did not rate as very important in evolutionary distinctiveness including species of deer mice and gerbils. While many criteria affect conservation decisions, preserving phylogenetic diversity provides an objective way to protect the full range of diversity generated by evolution.
CC Licensed Content, Shared Previously, Included in Evolutionary Patterns
- Biology 2e. Authors: Mary Ann Clark, Matthew Douglas and Jung Choi. Provided by: OpenStax CNX. Located at: Biology 2e. License: CC BY: Attribution 4.0.
- Biology and the Citizen. Author: Colleen Jones. Provided by: Pressbooks. Located at: Biology and the Citizen – Simple Book Publishing License: CC BY 4.0.
- An Interactive Introduction to Organismal and Molecular Biology, 2nd ed. Author: Andrea Bierema. Provided by: Pressbooks. Located at: An Interactive Introduction to Organismal and Molecular Biology, 2nd ed. – Simple Book Publishing. License: CC BY-NC-SA 4.0
- Harris, S.R. et al. 2010. Evolution of MRSA during hospital transmission and intercontinental spread. Science 327:469–474. ↵
- Isaac NJ, Turvey ST, Collen B, Waterman C, Baillie JE (2007) Mammals on the EDGE: Conservation Priorities Based on Threat and Phylogeny. PLoS ONE 2(3): e296. doi:10.1371/journal.pone.0000296 ↵