Variation in Populations
Section Goals
By the end of this section, you will be able to do the following:
- Describe the sources of variation in a population
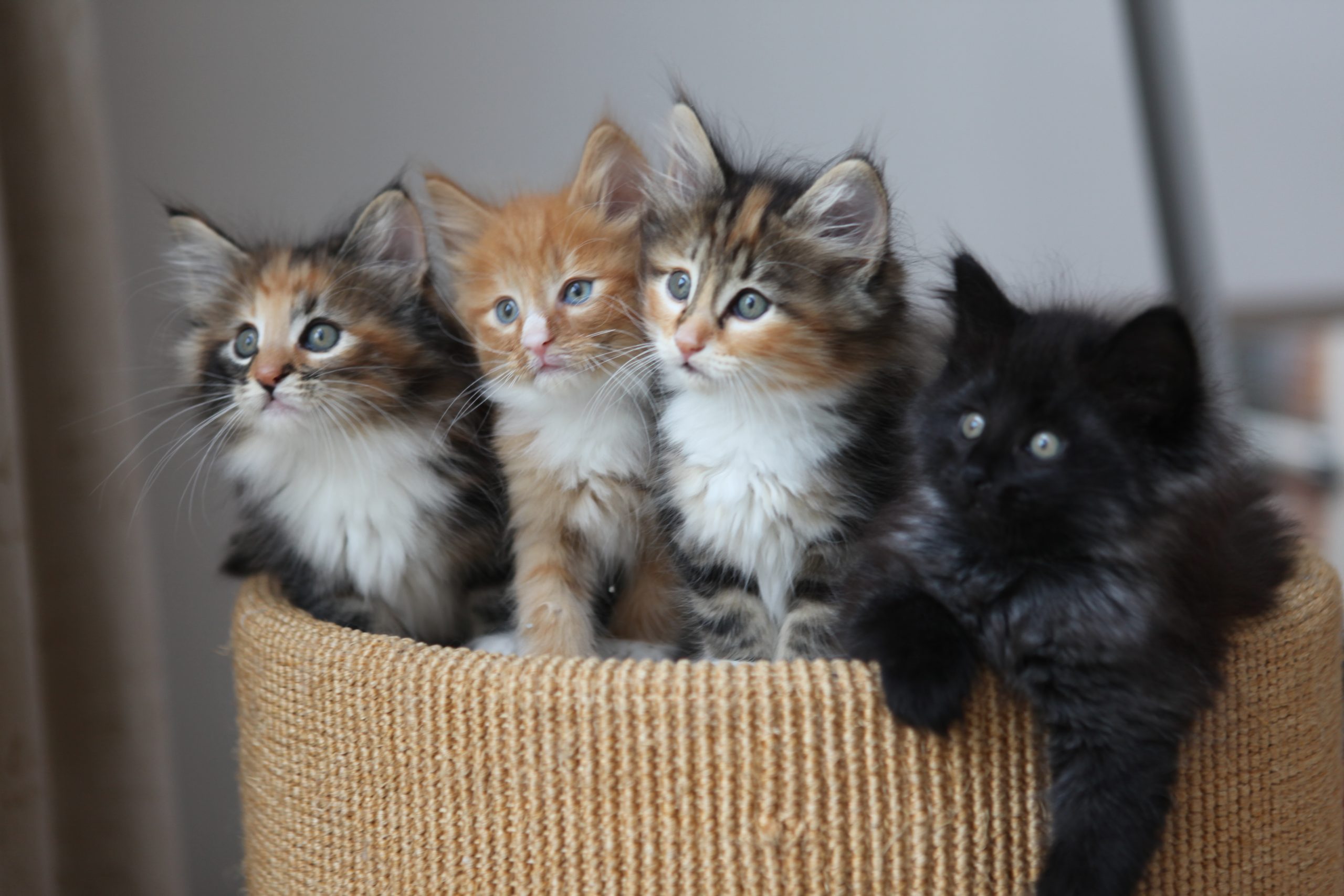
A population’s individuals often display different phenotypes, or express different alleles of a particular gene, which scientists refer to as polymorphisms. We call populations with two or more variations of particular characteristics polymorphic. A number of factors, including the population’s genetic structure and the environment (Figure 1) influence population variation, the distribution of phenotypes among individuals. Understanding phenotypic variation sources in a population is important for determining how a population will evolve in response to different evolutionary pressures.
Heritability is the fraction of phenotype variation that can be attributed to genetic differences, or genetic variance, among individuals in a population. The greater the heritability of a population’s phenotypic variation, the more susceptible it is to the evolutionary forces that act on heritable variation.
The diversity of alleles and genotypes within a population is called genetic diversity, or genetic variation. Genetic diversity in a population comes from two main mechanisms: mutation and sexual reproduction.
Mutations
Mutations are changes to an organism’s DNA, and are an important driver of new alleles, or new genetic variation in any population. An organism’s DNA affects how it looks, how it behaves, its physiology — all aspects of its life. So a change in an organism’s DNA can cause changes in all aspects of its life.
Species evolve because of mutations accumulating over time. The appearance of new mutations is the most common way to introduce novel genotypic and phenotypic variance. The genetic changes that mutation causes can have one of three outcomes on the phenotype:
- A mutation affects the organism’s phenotype in a way that is harmful or gives it reduced fitness—lower likelihood of survival or fewer offspring. These mutations are often quickly eliminated from the population by natural selection.
- A mutation may produce a phenotype with a beneficial effect on fitness, and will spread through the population.
- Many mutations will also have no effect on the organism’s fitness and can linger, unaffected by natural selection, in the genome. We call these neutral mutations.
Mutations may also have a whole range of effect sizes on the organism’s fitness that expresses them in their phenotype, from a small effect to a great effect.
Mutations can be beneficial, neutral, or harmful for the organism, but mutations do not “try” to supply what the organism “needs.” In this respect, mutations are random — whether a particular mutation happens or not is unrelated to how useful that mutation would be.
Not all mutations matter to evolution. Since all cells in our body contain DNA, there are lots of places for mutations to occur; however, not all mutations matter for evolution. Somatic mutations occur in non-reproductive cells and so won’t be passed onto offspring. For example, the yellow color on half of a petal on the red tulip in Figure 2 was caused by a somatic mutation. The seeds of the tulip do not carry the mutation. Cancer is also caused by somatic mutations that cause a particular cell lineage (e.g., in the breast or brain) to multiply out of control. Such mutations affect the individual carrying them but are not passed directly on to offspring.
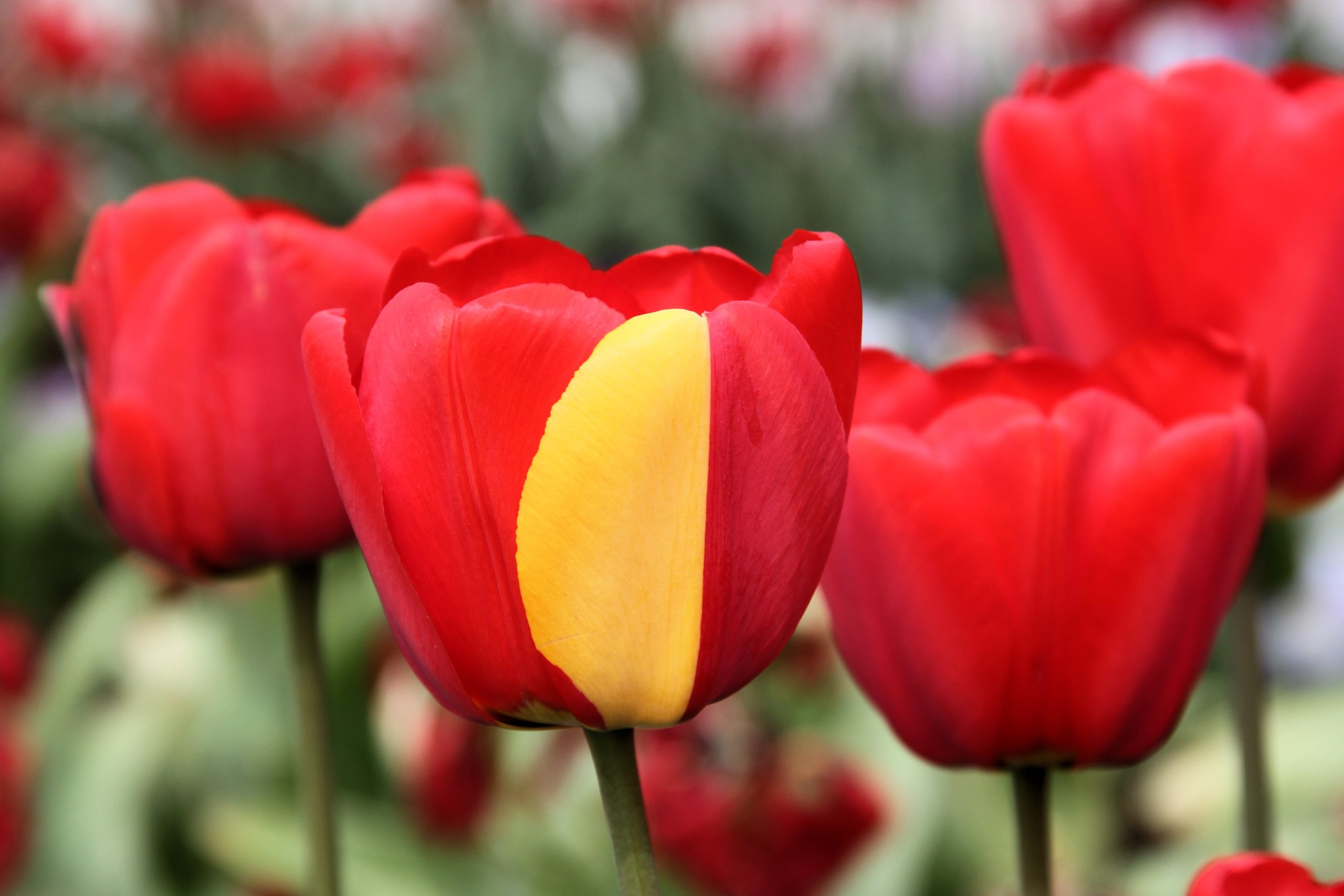
The only mutations that matter for the evolution of life’s diversity are those that can be passed onto offspring. These occur in reproductive cells like eggs and sperm and are called germline mutations.
Read more about how mutations are random and the famous Lederberg experiment that demonstrated this. Or read more about how mutations factored into the history of evolutionary thought. Or dig into DNA and mutations in this primer.
Sex and genetic shuffling
Sexual reproduction also leads to genetic diversity: Sex can introduce new gene combinations into a population and is an important source of genetic variation. When two parents reproduce, unique combinations of alleles assemble to produce the unique genotypes and thus phenotypes in each offspring.
You probably know from experience that siblings are not genetically identical to their parents or to each other (except, of course, for identical twins). That’s because when organisms reproduce sexually, some genetic “shuffling” occurs, bringing together new combinations of genes. For example, you might have bushy eyebrows and a big nose if one of your parents had genes associated with bushy eyebrows and the other parent had genes associated with a big nose (Figure 3).
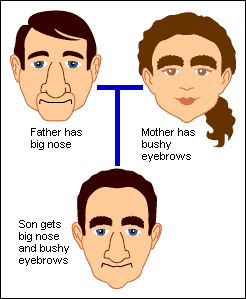
This shuffling is important for evolution because it can introduce new combinations of genes every generation. For example, in a particular population, plants with reddish flowers and plants with longer more tubular flowers might each do fine on their own – but if sex and genetic shuffling produced a plant with both traits (red tubular flowers), the combination might attract a new pollinator (hummingbirds) and alter the evolutionary trajectory of the lineage. Of course, sex and genetic shuffling can also break up good combinations of genes and form bad ones.
When scientists are involved in the breeding of a species, such as with animals in zoos and nature preserves, they try to increase a population’s genetic variance to preserve as much of the phenotypic diversity as they can. This also helps reduce the risks associated with inbreeding, the mating of closely related individuals, which can have the undesirable effect of bringing together deleterious recessive mutations that can cause abnormalities and susceptibility to disease. For example, a disease that is caused by a rare, recessive allele might exist in a population, but it will only manifest itself when an individual carries two copies of the allele. Because the allele is rare in a normal, healthy population with unrestricted habitat, the chance that two carriers will mate is low, and even then, only 25 percent of their offspring will inherit the disease allele from both parents. While it is likely to happen at some point, it will not happen frequently enough for natural selection to be able to swiftly eliminate the allele from the population, and as a result, the allele will be maintained at low levels in the gene pool. However, if a family of carriers begins to interbreed with each other, this will dramatically increase the likelihood of two carriers mating and eventually producing diseased offspring, a phenomenon known as inbreeding depression.
What about genes that impact development?
Development is the process through which a fertilized egg, the earliest stage of an embryo, becomes an adult organism. Throughout development, an organism’s genotype is expressed as a phenotype, exposing genes and the genetic elements that control their expression to the action of natural selection. Genetic variation in genes affecting development seems to have played an important role in evolution.
Changes in the genes controlling development can have major effects on the morphology (form and structure) of the adult organism. Because these effects are so significant, scientists suspect that changes in the expression of developmental genes have helped bring about major evolutionary transformations. Developmental changes, as well as new genes, may help explain, for example, how some hoofed mammals evolved into ocean-dwellers, how water plants invaded the land, and how small, armored invertebrates evolved wings.
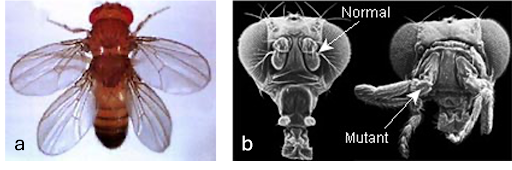
Developmental processes may also constrain the sorts of phenotypes that genetic variation can lead to, and so might prevent certain characters from evolving in certain lineages. For example, development may help explain why there are no truly six-fingered tetrapods among living species.
Environmental Variance
Genes are not the only players involved in determining population variation. Other factors, such as the environment also influence phenotypes. A beachgoer is likely to have darker skin than a city dweller, for example, due to regular exposure to the sun, an environmental factor. For some species, the environment determines some major characteristics, such as sex (Figure 5). For example, some turtles and other reptiles have temperature-dependent sex determination (TSD). TSD means that individuals develop into males if their eggs are incubated within a certain temperature range, or females at a different temperature range.
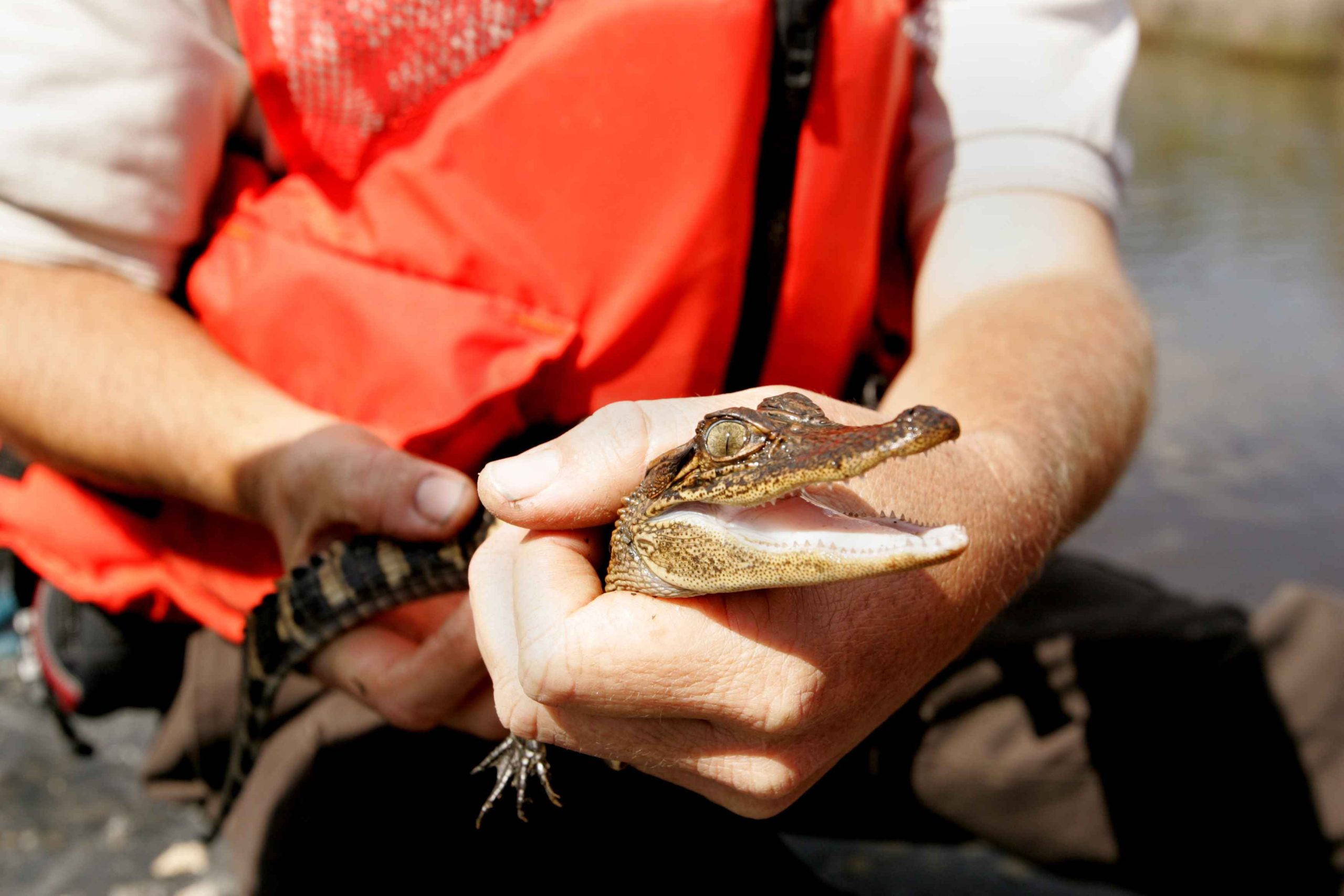
Geographic separation between populations can lead to differences in the phenotypic variation between those populations. We see such geographical variation between most populations and it can be significant. We can observe one type of geographic variation, a cline, as given species’ populations vary gradually across an ecological gradient. Species of warm-blooded animals, for example, tend to have larger bodies in the cooler climates closer to the earth’s poles, allowing them to better conserve heat. This is a latitudinal cline. Alternatively, flowering plants tend to bloom at different times depending on where they are along a mountain slope. This is an altitudinal cline.
If there is gene flow between the populations, the individuals will likely show gradual differences in phenotype along the cline. Restricted gene flow, alternatively, can lead to abrupt differences, even speciation.
CC Licensed Content, Shared Previously, Included in Mechanisms of Evolution
- Biology 2e. Authors: Mary Ann Clark, Matthew Douglas and Jung Choi. Provided by: OpenStax CNX. Located at: Biology 2e. License: CC BY: Attribution 4.0.
- Biology for Majors II. Authors: Shelly Carter and Monisha Scott. Provided by: Lumen Learning. Located at: Biology for Majors II | Simple Book Production. License: CC BY: Attribution 4.0.
- Adaptation. Provided by: UC Museum of Paleontology Understanding Evolution. Located at: https://evolution.berkeley.edu/evolution-101/mechanisms-the-processes-of-evolution/adaptation. License: CC BY-NC-SA 4.0
- Development. Provided by: UC Museum of Paleontology Understanding Evolution. Located at: https://evolution.berkeley.edu/evolution-101/mechanisms-the-processes-of-evolution/development/. License: CC BY-NC-SA 4.0
- Mutations. Provided by: UC Museum of Paleontology Understanding Evolution. Located at: https://evolution.berkeley.edu/evolution-101/mechanisms-the-processes-of-evolution/mutations/. License: CC BY-NC-SA 4.0
- Sex and Genetic Shuffling. Provided by: UC Museum of Paleontology Understanding Evolution. Located at: https://evolution.berkeley.edu/evolution-101/mechanisms-the-processes-of-evolution/sex-and-genetic-shuffling/. License: CC BY-NC-SA 4.0