Chapter 7: Development of the Brain and Nervous System
7.4: Neuroplasticity
Anytime you learn something new, the structure of your brain physically changes. The brain’s ability to reorganize or “rewire” its connections in response to intrinsic or extrinsic experiences is called neuroplasticity.
Learning depends on the plasticity of the circuits in the brain and the ability to make lasting changes in synaptic transmission (Clark et al., 2018; “The Brain from Top to Bottom”, n.d.). The brain can thus be said to store information in networks of modified synapses, the arrangement of which constitutes the information. By activating these synaptic networks, the brain is able to retrieve the stored information.
Our understanding of the rules that govern the networking of neurons goes back to the groundbreaking work by Donald Hebb over 70 years ago. Hebb proposed that if two neurons are active at the same time, the synapses between them are strengthened—this is captured in the famous phrase “Neurons that fire together, wire together.” This hypothesis inspired many researchers, and the first mechanism supporting it, long-term potentiation (LTP), was discovered a few decades later in the early 1970s.
Long-term potentiation (LTP) is a persistent strengthening of a synaptic connection (Clark et al., 2018). LTP is based on the Hebbian principle: cells that fire together wire together. There are various mechanisms, none fully understood, behind the synaptic strengthening seen with LTP. One known mechanism involves a type of postsynaptic glutamate receptor, called NMDA (N-Methyl-D-aspartate) receptors. These receptors are normally blocked by magnesium ions; however, when the postsynaptic neuron is depolarized by multiple presynaptic inputs in quick succession (either from one neuron or multiple neurons), the magnesium ions are forced out, allowing calcium (Ca2+) ions to pass into the postsynaptic cell. Next, Ca2+ ions entering the cell initiate a signaling cascade that causes a different type of glutamate receptor, called AMPA (α-amino-3-hydroxy-5-methyl-4-isoxazolepropionic acid) receptors, to migrate from within the postsynaptic cell to the cell membrane and insert into the postsynaptic membrane. This is relevant to neuroplasticity, since activated AMPA receptors allow positive ions to enter the cell. So, the next time glutamate is released from the presynaptic neuron, it will have a larger excitatory effect (EPSP) on the postsynaptic cell because the binding of glutamate to these newly available AMPA receptors will allow more positive ions into the cell. The insertion of additional AMPA receptors on the cell membrane strengthens the synapse and means that the postsynaptic neuron is more likely to fire in response to presynaptic neurotransmitter release. Some drugs of abuse co-opt the LTP pathway, and this synaptic strengthening can lead to addiction.
In addition to LTP strengthening synaptic connections, neuroplasticity also requires weakening of some connections. One well-studied process underlying the weakening of synaptic connections is Long-Term Depression (LTD). Long-term depression is essentially the reverse of LTP. Similar to long-term potentiation, long-term depression also involves AMPA receptors. In this situation, calcium that enters through NMDA receptors initiates a different signaling cascade, which results in the removal of AMPA receptors from the postsynaptic membrane (Figure 4). The decrease in AMPA receptors in the membrane makes the postsynaptic neuron less responsive to glutamate released from the presynaptic neuron. While it may seem counterintuitive, LTD may be just as important for learning and memory as LTP. The weakening and pruning of unused synapses allows for unimportant connections to be lost and makes the synapses that have undergone LTP that much stronger by comparison.
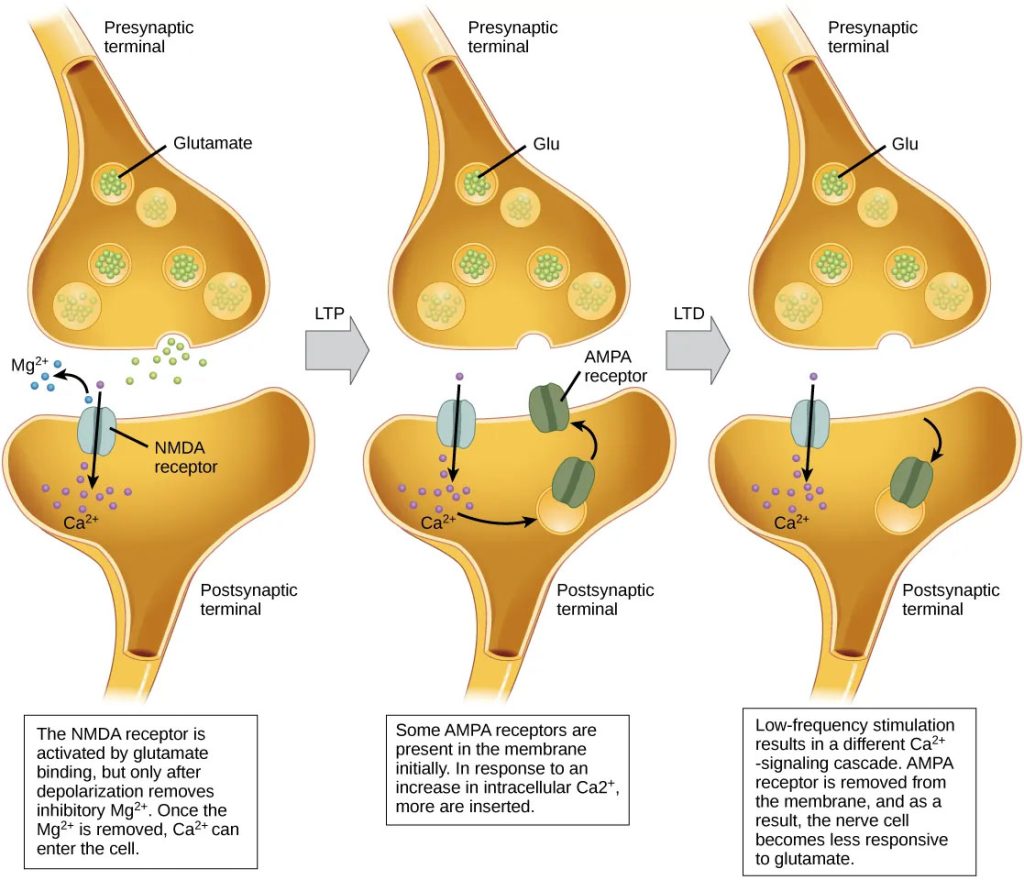
Even today, Hebb’s rule, as it is often known, remains one of the primary factors for predicting which synapses will be strengthened in a network of neurons. More recent research has uncovered other characteristics of the networking of groups of neurons. For example, the LTP that leads to synaptic strengthening is very specific to neurons that are activated simultaneously, and only to such neurons.
Text Attributions
This section contains material adapted from:
Clark, M. A., Douglas, M., & Choi, J. (2018). 35.2 How Neurons Communicate. In Biology 2e. OpenStax. Access for free at https://openstax.org/books/biology-2e/pages/35-2-how-neurons-communicate License: CC BY 4.0 DEED.
Duboc, B. (2002). The Brain From Top To Bottom: Memory and the Brain: Plasticity in Neural Networks. Access for free at https://thebrain.mcgill.ca/ License: CC (Copyleft).
Media Attributions
- Long-term Depression (LTD) © Openstax is licensed under a CC BY-NC-SA (Attribution NonCommercial ShareAlike) license
A persistent strengthening of synaptic connections based on recent patterns of activity. A mechanism underlying “neurons that fire together, wire together.”
A long lasting decrease in the strength of synaptic connection based on recent patterns of activity.