4.4: Tools of Cognitive Neuroscience – EEG and MEG
Advances in technology have led to increasingly sophisticated brain recording techniques. Just as X-rays allow us to peer inside the body, neuroimaging techniques enable us to view the working brain (Raichle, 1994). Biopsychology employs various noninvasive methods to study human brain function, each with its own advantages and disadvantages. It’s crucial to recognize that each technique offers a unique perspective on brain activity. Understanding these methods is essential, as our comprehension of the brain is closely tied to available technologies. As new techniques develop, we can anticipate an even deeper understanding of brain function (Biswas-Diener, 2023).
Electroencephalography (EEG) measures the electrical activity of the brain and has been used for a century (e.g., Berger, 1929). When large populations of neurons are active, they create a small electrical voltage that passes through the skull and scalp. Electrodes on the participant’s head pick up the voltages, which are amplified and recorded. Researchers can record the voltages from brain activity as the participant performs a task.
The electrical signals generated in the brain become distorted as they travel through brain tissue, skin, and bone. As a result, researchers can only approximate the original source of these signals within the brain. This uncertainty is especially pronounced for signals originating deep within the brain (even with advanced tools such as structural brain scans, high-density electrode coverage from a cap with 256 electrodes, and sophisticated analysis algorithms for localization). Thus, EEG’s spatial resolution—its ability to pinpoint where activity occurs in the brain—is relatively low. Conversely, EEG’s temporal resolution is excellent and indicates when something happens in the brain with millisecond precision. EEG’s superior temporal resolution makes it ideal for examining the brain’s rapid response to a stimulus event, or the event-related potentials (ERP). In a typical ERP experiment, researchers might play a visual or auditory event like a word, and measure the corresponding voltage changes that unfold in the brain over the next few hundred milliseconds. The amplitude, timing, and topography (position) of the EEG signal capture the underlying neural/mental processes.
The high temporal resolution and continuous recording of EEG allows it to capture different frequency brain waves, such as theta waves (oscillating at 4-7 Hertz (Hz), i.e., cycles per second), alpha waves (at 8-13 Hz), and beta waves (at 14-30 Hz). Brain oscillations, reflecting the combined activity of millions of neurons, capture attentional and conscious states. These oscillations play a crucial role in information encoding and attention modulation in the brain (da Silva, 2013). In addition to research applications, EEG plays a crucial role in clinical diagnosis, particularly in identifying epilepsy, seizures, and sleep disorders.
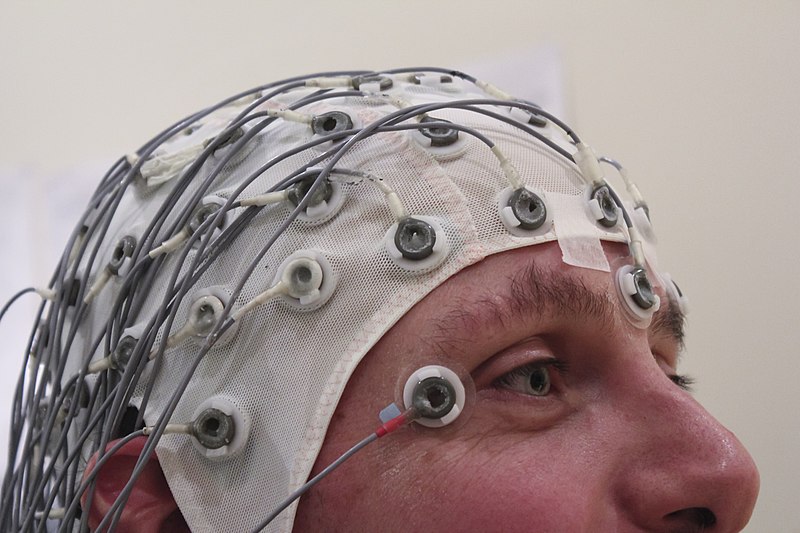
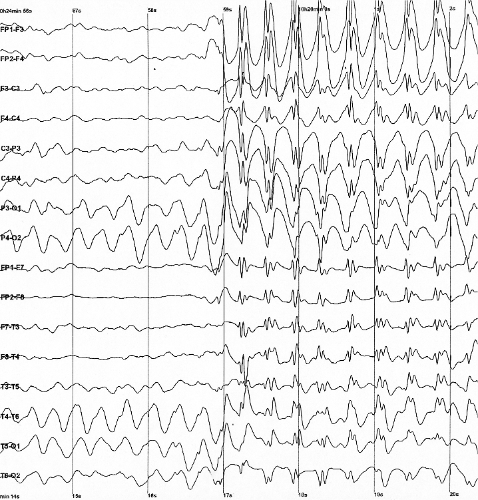
Magnetoencephalography (MEG) is similar to EEG, but instead of electrical signals, MEG picks up the weak magnetic fields generated by the flow of electrical charge associated with neural activity. Because the magnetic fields generated by brain activity are so small, special rooms are needed that shield out magnetic fields in the environment so that the MEG sensors pick up magnetic fields from neural activity without environmental contamination. Similar to EEG, MEG also has excellent (millisecond) temporal resolution. The spatial resolution of MEG is far better than EEG because magnetic fields pass relatively unchanged through hard and soft tissue, so are not distorted by skull and scalp. Despite MEG’s excellent spatial and temporal resolution, it is less widely used than EEG because its much more expensive and complex to operate.
Text Attributions
This section contains material adapted from:
Biswas-Diener, R. (2023). The brain and nervous system. In R. Biswas-Diener & E. Diener (Eds), Noba textbook series: Psychology. Access for free at http://noba.to/4hzf8xv6 License: CC BY-NC-SA 4.0 DEED
Media Attributions
- EEG Recording Cap © Wikimedia is licensed under a CC BY-SA (Attribution ShareAlike) license
- Spike Waves © Wikimedia is licensed under a CC BY-SA (Attribution ShareAlike) license
A neuroimaging technique that measures electrical brain activity via multiple electrodes on the scalp.
A term that refers to how small the elements of an image are; high spatial resolution means the device or technique can resolve very small elements; in neuroscience, it describes how small a structure in the brain can be imaged.
A term that refers to how small a unit of time can be measured; high temporal resolution means capable of resolving very small units of time; in neuroscience, it describes how precisely in time a process can be measured in the brain.
A brain science technique that measures brain activity by picking up the tiny magnetic fields generated by neural activity.