Allele function: Why are phenotypes dominant or recessive?
The wild-type phenotype is often the result of fully functional alleles of a gene, while mutant phenotypes arise due to alterations in the function of one or more proteins. The variant alleles are often described in terms of how they affect protein function: loss-of-function alleles reduce the function of a protein (or sometimes make no functional protein at all). Gain-of-function alleles do something extra: perhaps a greater quantity of protein is produced, or a protein that is normally only active under certain circumstances is active in additional circumstances.
Loss-of-function mutations are usually (but not always) recessive mutations: having a back-up fully functional allele means that the organism still has some of the protein available. For example, the white eye color of certain mutant fruit flies is a recessive phenotype. It is due to a loss-of-function in a protein that contributes to pigment production.
Gain-of-function alleles often (but not always) result in a dominant phenotype. When an allele does something extra, the back-up copy can’t block that extra function.
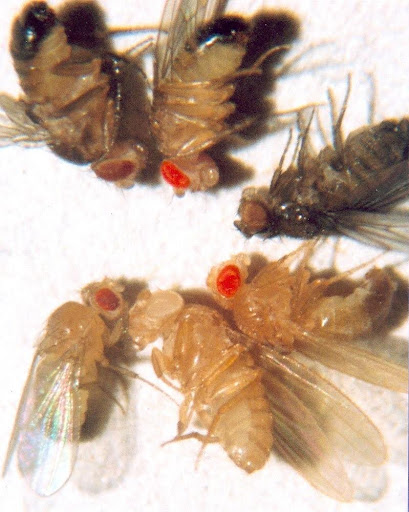
As an analogy, if you are making dinner but one of the burners of your stove is broken (a loss of function), you can still make dinner on another burner. The broken burner does not affect your dinner. But if all of the burners were broken – akin to homozygous loss-of-function mutations – your dinner will not be cooked. A gain of function mutation would be a burner that can’t be shut off: Your kitchen might be in danger of catching fire, regardless of whether the other burners are functioning properly.
For the example of eye color mutations in the fruit fly: as long as there is one functional copy of the enzymes needed to produce eye pigments, eye pigments are produced and the fly’s eyes are red. A homozygous mutation that knocks out the function of one protein blocks all pigment production, resulting in eyes that are white (Figure 1).
An example of a gain-of-function mutation is seen in the antennapedia gene (Antp) in fruit flies. Expression of the Antp gene triggers the development of leg structures. The gene is not normally expressed in the head of the insect – there is no antp protein in those cells of the body. However, Antp mutant flies mis-express the gene, so the protein is produced in embryonic cells that would normally develop into antenna. In these fruit flies, mis-expression of the antp gene causes leg-like structures to grow where wild-type flies have antenna[1]! A fly with this phenotype is shown in Figure 2. The extra production of the Antp protein makes it a gain of function mutation, and the antenna-leg phenotype is dominant. (Note: This is not a normal variation seen in wild fruit fly populations! This is a phenotype studied in the lab.)
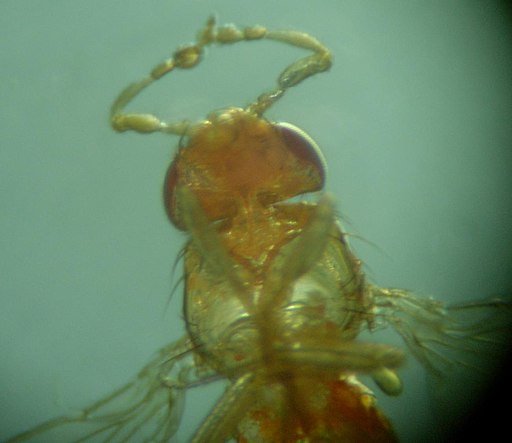
Although most gain-of-function alleles contribute to dominant phenotypes, and most loss-of-function alleles contribute to recessive phenotypes, there are exceptions. Haploinsufficient genes are one example. Haploinsufficiency means that one functional copy of the gene is not enough to produce a normal phenotype. Haploinsufficient genes are often genes for which the quantity of the protein product is important. For haploinsufficient genes, loss-of function alleles may be dominant because there is not enough protein produced to get the job done.
Test Your Understanding
So far in this text, we’ve considered dominance and recessiveness as if they are the only options for alleles. A diploid individual can only have two alleles, but in biological populations, when all individuals are accounted for, there may be far more than just two different variations of each gene. Those alleles can interact with each other in far more ways than simple dominance or recessiveness. Some of those types of interactions are described in the next sections and are summarized in Table 1.
Terminology | Definition | Characteristic offspring ratios | Example |
Incomplete dominance | Heterozygotes display a phenotype intermediate to that of the homozygous dominant and recessive | A monohybrid cross gives a 1:2:1 phenotypic offspring ratio, since the heterozygote displays a different phenotype than the homozygous dominant | Red snapdragon (flowers) crossed with white snapdragons give pink offspring, which are always heterozygous. Crossing two pink carnations gives a 1:2:1 ratio of red:pink:white offspring. |
Codominance | Both the dominant and recessive phenotypes are displayed in the heterozygote | A monohybrid cross gives a 1:2:1 phenotypic offspring ratio, since the heterozygote displays a different phenotype than the homozygous dominant | Human ABO bloodtypes. Parents of blood type A and blood type B can have children with bloodtype AB. |
Incomplete penetrance | The phenotype is not always as expected from the genotype | The ratio of offspring affected by the trait is lower than expected | 70% of Individuals with BRCA1 mutations get breast cancer, but not all |
Variable expressivity | Individuals with the same genotype may vary in the extent to which a phenotype is expressed. | Ratios are as expected for a dominant or recessive allele, but phenotypes vary. | Polydactyly can vary in the number of extra digits. |
Pleiotropy | Pleiotropic alleles contribute to multiple phenotypes | Ratios are as expected, but multiple traits appear linked together | Patients with mutant ATM alleles have ataxia, telangiectasia, immune dysfunction, and high rates of cancer |
Recessive lethal allele | Individuals homozygous for a recessive lethal allele are never seen in the population, because those individuals die during embryonic or fetal development. | 2:1 ratio of offspring phenotypes | Tailless Manx cats are always heterozygous. Crossing two Manx cats gives 2:1 ratio of tailless to tailed kittens. T |
Media Attributions
- Antennapedia © Wikipedia is licensed under a CC BY-SA (Attribution ShareAlike) license
- Struhl, G. A homoeotic mutation transforming leg to antenna in Drosophila. Nature 292, 635–638 (1981). ↵