How do we know? Determining the structure of DNA
DNA is the genetic material
Determining the structure of DNA involved many researchers and the work of several decades.
In the first half of the 20th century, there was considerable debate about the nature of the genetic material. Chromosomes were visible inside cells and were known to be hereditary units, but chromosomes contain both DNA and protein elements. It was known that both DNA and proteins were long polymers built from smaller building blocks. But how those building blocks were assembled was not known. Proteins, built from twenty different amino acids, were chemically far more complex than DNA, which contained only four different nucleotides, so most scientists at the time believed that protein would eventually be shown to be the genetic material.
A series of landmark experiments helped to convincingly determine that DNA was the genetic material. The works of Oswald Avery, Colin Macleod and Maclyn McCarty, and Alfred Hershey and Martha Chase were among these important contributions, but their work all depended on data from earlier studies.
Avery, Macleod, and McCarty, for example, built on earlier work by Frederick Griffith. Avery, Macleod, and McCarty showed that DNA from some bacteria – but not protein or RNA – could transform the characteristics of other bacteria. Thus, DNA passed genetic information from one organism to another. Their work was published in 1944; however, their conclusions were not universally accepted. Critics maintained that their experimental design might allow protein contamination in their DNA samples, thus not completely ruling out protein as the genetic material.
The work of Alfred Hershey and Martha Chase, published in 1951, provided additional evidence that DNA is the genetic material. Hershey and Chase were working with bacteriophages, which are viruses that infect bacteria. Hershey and Chase used radioactively labeled protein and DNA to show that only the DNA of the virus – and not its protein – is transferred to a host bacterium upon infection. Because the transferred DNA is sufficient for the virus to replicate in the host cell, producing more virus, it could be concluded that the genetic material of the virus was DNA.
As more evidence accumulated for DNA as the genetic material, other researchers were attracted to the study of DNA. By the late 1040s and early 1950s, many scientists were actively working to discover the structure of DNA. James Watson and Francis Crick were probably the most well-known of the researchers. However, like most scientific discoveries, the full history included work from many other scientists, all of whom were also, in turn, influenced by the work of other scientists.
Erwin Chargaff, for example, showed that for any DNA sample, the ratios of adenine and thymine were always equal, as were the ratios of thymine and cytosine. These equivalences became known as “Chargaff’s rules”, and we know now that Chargaff’s rules are true because A and T are paired within the double helix, as are C and G.
These landmark experiments greatly contributed to the scientific environment of the 1950s. Because DNA was so chemically simple with only four nucleotide building blocks, it was difficult to understand how it could function as the genetic material. So the next big question to answer was how nucleotides were assembled. Understanding the structure would give a glimpse into how DNA functions.
Although many research groups were racing to solve this problem, two different methods were primarily used. These methods were X-ray diffraction and model-building. Both grew directly out of work from protein physicists – remember that protein was the favored molecule in the early 1900’s, so a lot of work had already been done in that field.
Protein Structure: Linus Pauling, Robert Corey, and Herman Branson
In many ways, the story could begin with Linus Pauling. Pauling was a chemist who used quantum mechanics to study chemical bonding. His research interests were quite varied: over the course of his career, Pauling published research on chemical bonding and structure, the structure of biomolecules, explosives and weaponry, sickle-cell anemia, and the health benefits of vitamin C. for which he ultimately was awarded the Nobel Prize in Chemistry. Linus Pauling and his wife Ava, a human rights activist, are shown in the photograph in Figure 9.
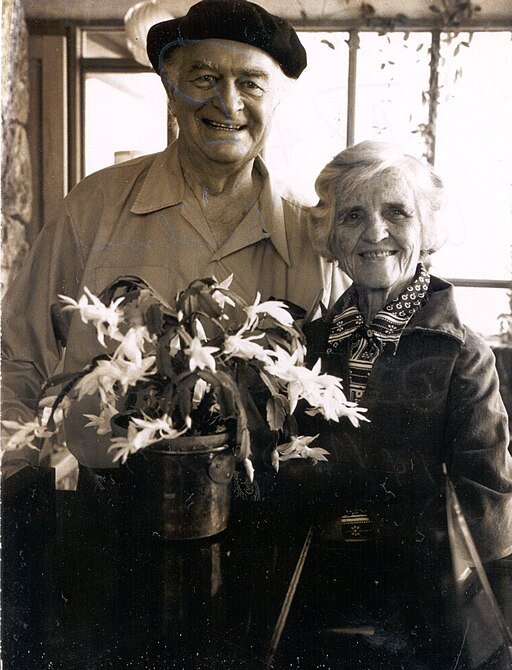
Over a long career, Linus Pauling studied chemical bonding and structure, biomolecules, explosives and weaponry, sickle-cell anemia, and vitamin C. He has the unique honor of having won two unshared Nobel Prizes: one for Chemistry in 1954, and the Nobel Peace Prize in 1962. Linus Pauling’s life was not without controversy: Early years of his career coincided with the rise of Naziism and the Second World War, and he worked extensively on explosives and weaponry at the beginning of the Second World War. He was invited to head the Manhattan Project, the classified government program that ultimately led to the development of the atomic bomb, although he turned down the offer. In later years, Pauling rethought his stance on war and became an ardent pacifist, advocating against nuclear weapons. It was this work that lead to his 1962 Nobel Peace prize. His wife, Ava Pauling, was also a prominent human rights activist. Pauling’s activism led to his condemnation as a communist during the Cold War, and for several years his passport was revoked and he was prohibited from international travel. Pauling’s work on pacifism eventually led him to leave in 1964 his academic “home” of over four decades, the California Institute of Technology, in response to pressure from university leadership. Despite this, Pauling continued his work in chemistry (at University of California,San Diego and Stanford University) and his humanitarian work (advocating against military action in Vietnam, Cuba and Nicaragua, and the Persian Gulf).
By the late 1940s, Pauling had shifted his research focus from chemical bonding and structure to larger, more complex biomolecules, particularly proteins. Remember, protein had not yet been ruled out as the genetic material.
Like DNA, proteins are complex polymeric molecules. They are built as long chains of amino acid monomers. The chains fold into complex shapes. Pauling was interested in the chemical bonds that drive the folding of the chains.
To study these complex structures, Pauling employed the technique of X-ray diffraction. Briefly, this technique works by shining X-rays through an ordered crystal of protein (under proper conditions, proteins can form crystals much salt can). The X-rays do not pass straight through the crystal. Instead, they are bounced aside or diffracted if they encounter an atom within the crystal. The diffracted beams are captured through photographic film, much like taking a picture by capturing light via a digital camera sensor. The diffraction pattern can be used to mathematically back-calculate the arrangement of the atoms in the crystal, determining the protein structure.
The technique of X-ray diffraction is difficult and involves complex mathematics, and in the mid-1930s, Pauling did not have the equipment or experience for this work. So, Pauling eventually recruited two additional experts in X-ray diffraction: Robert Corey and Herman Branson.
Robert Corey had done preliminary work using X-ray diffraction to determine the structure of biological molecules. He joined Pauling’s lab at Caltech in 1937. While working with Pauling, Corey initially worked to determine the amino acid structure of several amino acids. During World War II, Corey worked to administer Pauling’s research collaborations with the War Department, returning to the study of biological molecules in the late 1940s.
In 1948-1949, Pauling recruited Herman Branson (Figure 10), a visiting researcher from Howard University with experience in X-ray diffraction and mathematical chemistry. By that point, Pauling and Corey had data regarding many features of protein structure, including the structure of several individual amino acids and, notably, the planar (flat) shape of the peptide bond linking adjacent amino acids. With these preliminary data as constraints, Branson was tasked with calculating all possible protein structures that could be consistent with the existing information and the X-ray diffraction patterns. He came up with two, including the alpha-helical structure which is one of two main secondary structures found in proteins, and Pauling, Corey, and Branson published the work in Proceedings of the National Academy of the Sciences (PNAS) in 1951. Later X-ray diffraction data from other labs confirmed Branson’s calculations.
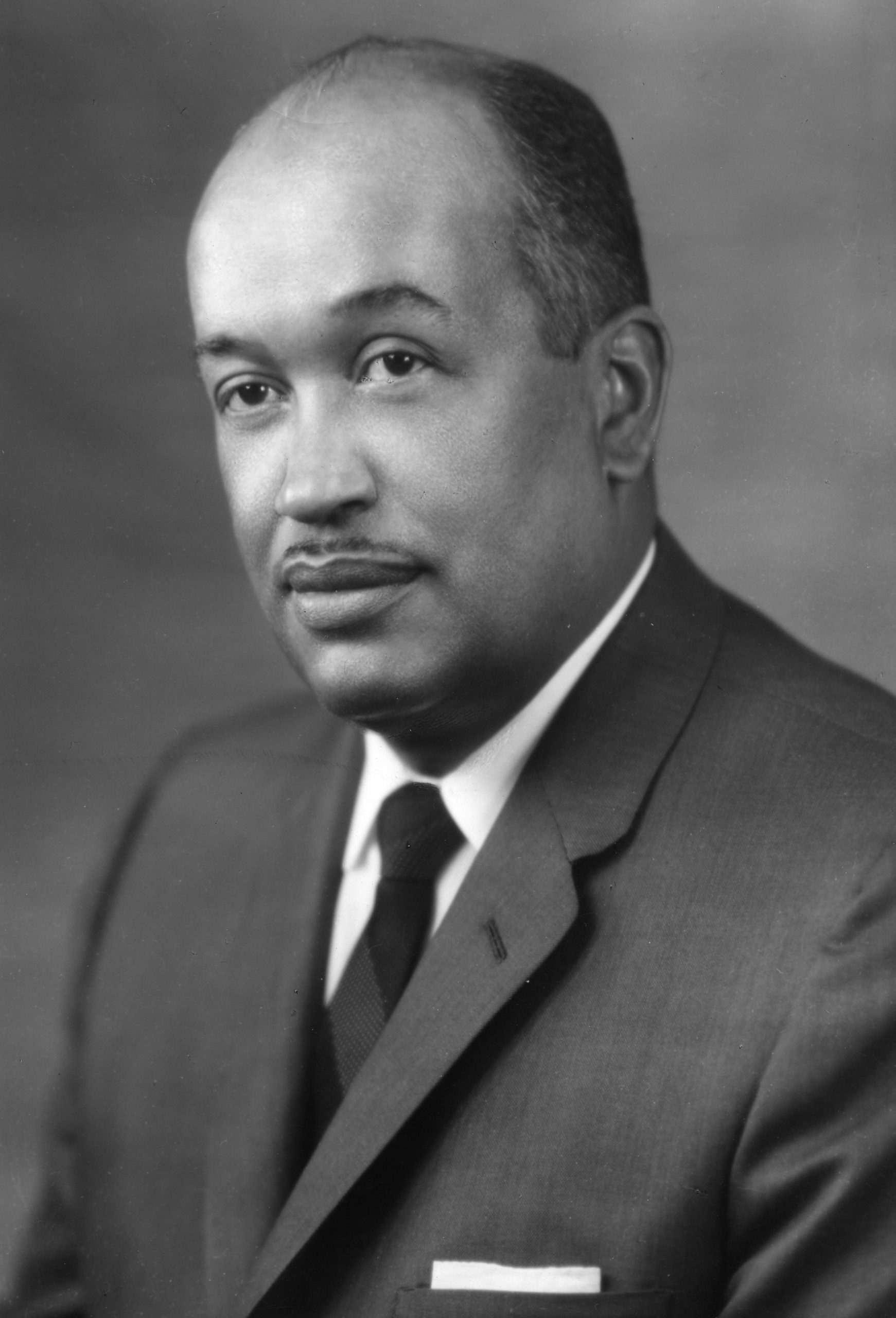
Who contributed? Who gets the credit? In 1954, Linus Pauling was awarded an individual Nobel Prize for his work “for his research into the nature of the chemical bond and its application to the elucidation of the structure of complex substances’. Although up to three researchers may share a Nobel Prize, it is notable that this was an unshared award (Pauling’s first of two unshared Nobel Prizes). This is, however, uncertainty regarding the role Pauling, Branson, and Corey played in this project, with evidence of disagreement among the collaborators. In later years Branson asserted that his contribution was much larger than his authorship on the PNAS paper suggested. While Branson’s work was limited to the one-year project that led to proposing the alpha helix structure, certain inconsistencies between the X-ray diffraction data, Pauling’s assumption regarding the water content of the helix, and Branson’s measurements worried Pauling. [ref http://scarc.library.oregonstate.edu/coll/pauling/proteins/narrative/page33.html]. It was not until his scientific competitors appeared to be closing in that Pauling consented to publish the structures resulting from Branson’s calculations – which, as history has shown, have proven largely correct. [https://www.pnas.org/doi/10.1073/pnas.2034522100#body-ref-ref7 ] Branson went on to hold prestigious positions in academia, acting as President of two different institutions: Central State University in Ohio, and Lincoln University in Pennsylvania.
In addition to X-ray diffraction, Pauling’s lab relied heavily on what came to be known as “model-building”. Essentially, X-ray diffraction allowed calculation of certain bond angles, bond lengths, and geometries within larger molecules, much like observing the shape of a puzzle piece without knowing what the full puzzle looks like. Then, through a bit of trial and error, Pauling and his colleagues would figure out how the pieces could physically fit together, making sure that their final proposed structure was consistent with the existing data. Model building was remarkably successful for determining features of protein structure: after the alpha helix collaboration with Branson, Pauling and Corey went on to publish papers describing the beta sheet and other features of protein structure.
When the work of researchers like Avery, Macleod, McCarthy, Hershey, and Chase added up to suggest that it was DNA, not protein, that was the genetic material, many researchers, including Pauling, turned their attention to determining the structure of DNA.
Model-building and X-ray diffraction: DNA structure
Using what he knew about the chemical composition of nucleotides, and with helices on his mind, Pauling proposed a helical structure for DNA as well. His structure was spectacularly wrong: he proposed a helix with three intertwined strands, with phosphates pointing inward to the center axis of the helix and bases extending outward. In this, he failed to address both Chargaff’s ratios of A:T and C:G equivalency, as well as the chemistry of acids: he used the uncharged, protonated structure of the nucleotides even though, as an acid, the phosphates of DNA are negatively charged at pH 7. Negatively charged phosphates clustered in the center of a helix would repel one another, making Pauling’s proposed model chemically impossible.
In Maurice Wilkins’ lab at King’s College, London, researcher Rosalind Franklin was using X-ray diffraction methods to understand the structure of DNA.
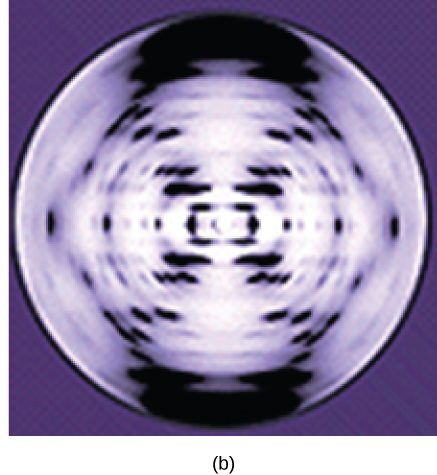
Rosalind Franklin’s X-ray diffraction methods involved shining a beam of X-rays through a sample of DNA, much like the X-ray diffraction patterns of protein crystals. As in protein diffraction, the X-ray beams bounce off of, or are diffracted by, atoms in the DNA structure. The pattern in which the beams are diffracted is determined by the arrangement of atoms in the molecule, so the structure can be determined from the diffraction pattern. One of Franklin’s diffraction images is shown in Figure 11. The X-shape that is clearly visible in this image was immediately suggestive of a helix, as was well-known from X-ray images of protein helices.
While Franklin labored at the tedious calculations required to determine structure from X-ray data, along came the team of James Watson and Francis Crick. Watson and Crick were model builders using the very methods that Pauling had embraced. Bringing together information from many researchers, including Chargaff’s rules for the ratios of the bases and, crucially, Franklin’s X-ray diffraction data and calculations, which they had access to unbeknownst to Franklin, Crick and Watson used model-building techniques to come up with a model in which all the data fit together.
Watson and Crick proposed the structure that we now accept to be correct: DNA is made up of two strands that are twisted around each other to form a right-handed helix. Base pairing takes place between a purine and pyrimidine on opposite strands so that A pairs with T and G pairs with C as suggested by Chargaff’s Rules. Thus, adenine and thymine are complementary base pairs, and cytosine and guanine are also complementary base pairs.
The final X-ray diffraction calculations from both Wilkins and Franklin provided the data to support Watson and Crick’s model. All three lab groups – Watson and Crick, Wilkins, and Franklin – published papers describing the structure of DNA, back-to-back-to-back in 1953 in the same issue of Nature.
Watson and Crick’s paper, the first of the three in the issue, provided a clear, modeled illustration of the B-form structure of DNA, which is the most common form in cells. The second paper was from Wilkins’ research group and described the structure of A-form DNA, which occurs under conditions of low humidity. The final paper was Franklin’s, which detailed the structure of B-form DNA as calculated from diffraction data. This paper was the only one of the three to provide experimental evidence for the B-form structure. In 1962, James Watson, Francis Crick, and Maurice Wilkins were awarded the Nobel Prize in Medicine. Franklin, who had died of ovarian cancer in 1958, was not eligible for recognition, as Nobel Prizes are not awarded post-humously.
Media Attributions
- Linus and Ava Pauling © Wikipedia is licensed under a CC BY-SA (Attribution ShareAlike) license
- X-ray image of DNA © LabXchange is licensed under a Public Domain license