Linkage and Mapping
Objectives
After completing this module, students will be able to:
- Define: linked vs unlinked genes, parental vs. recombinant classes of offspring, locus (plural loci), morph, polymorphism, haplotype
- Calculate recombination frequencies from offspring ratios and construct a genetic map using a two- or three-point testcross
- Use a pedigree to infer linkage
- Explain how tools of molecular genetics can be used in mapping
- Explain how genome association studies can be used to identify loci associated with a phenotype
Source Material
- Selected images and text modified from Open Genetics Lectures[1]. Chapter 37: DNA variation studied with microarrays
- Selected images and text remixed from Online Open Genetics (Nickle and Barrette-Ng), available through Biology LibreTexts[2]. Chapter 7: Linkage and mapping and Chapter 10: Molecular Markers and Quantitative Traits
Introduction
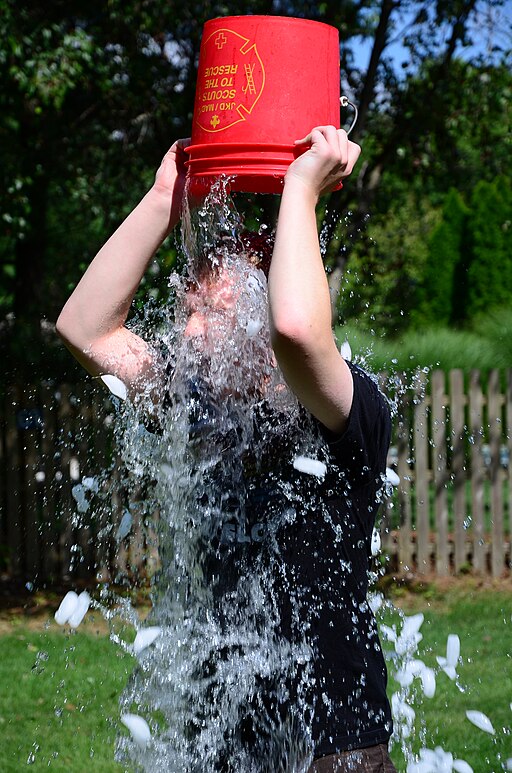
In the summer of 2014, the Ice Bucket Challenge (Figure 1) swept across the United States before spreading worldwide. Participants posted short videos of themselves pouring a bucket of ice water over their heads, challenging others to either do the same or donate to ALS research[3].
ALS stands for amyotrophic lateral sclerosis. It is also known as motor neuron disease and Lou Gehrig’s Disease, after the famous baseball player who developed the disease in 1939. ALS is a progressive neurodegenerative disease characterized by the loss of motor neurons. Motor neurons control the function of muscles, so ALS patients gradually lose muscle function. As the disease progresses, patients become paralyzed, without the ability to move, speak, or eat. In the final stages of the disease, patients lose their ability to breathe. Respiratory failure is the most common cause of death for ALS patients.
There is no cure for ALS: treatment can slow but not stop progression of the disease. Death usually comes within 2-5 years after initial diagnosis, although about 10% of ALS patients live longer than 10 years after diagnosis. The theoretical physicist Stephen Hawking (shown with his daughter, Lucy Hawking, in Figure 2) lived for more than 5 decades with a slow-progressing form of ALS.
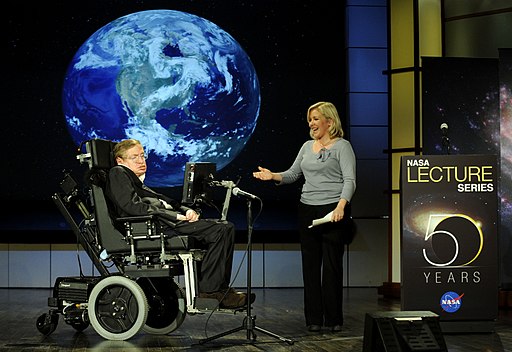
Most cases of ALS are sporadic, meaning that a newly diagnosed patient most likely has no family history or risk factors for the disease. But about 10% of ALS cases are familial: more than one member of the family can be affected by the disease. This indicates a genetic component to the disease. One important step in ALS research is therefore identifying causative genes: understanding the normal gene function and how variants cause disease can eventually lead to targeted treatments.
The Ice Bucket Challenge resulted in a windfall of about $115 million dollars to the ALS Association in the United States in the first six weeks after the challenge went viral. Similar organizations worldwide also benefitted. The ALS Association used much of these funds to support patients and their families and fund research. In 2015, the ALS Association awarded nearly four times the amount of research funds as in 2014. Within 18 months, funded researchers had identified a gene linked with susceptibility to ALS[4]. By 2019, five genes had been identified by researchers funded at least in part with Ice Bucket Challenge dollars, with at least one of these genes a target for a therapy in development.
So how are genes associated with a trait identified? Mendelian inheritance treats genes as an abstract concept – and, in fact, Mendel did not know that the patterns of heredity he described were a result of inheritance of physical chromosomes. However, today we understand genes to be information housed on molecules of DNA. The equal segregation and independent assortment that Mendel observed is due to the behavior of the physical chromosomes during meiosis.
This means that phenotypes that we study using genetic crosses and pedigrees can be mapped to specific parts of a chromosome, where we can analyze differences in DNA sequence to predict changes in function. We use the word “mapping” to describe the identification of parts of a chromosome that are associated with specific traits, and the word locus to describe the specific location on a chromosome.
We also use the work linkage to describe the relationship of a trait to a chromosome and the relationship of genes near to one another on a chromosome. You’ve seen this term used when we describe sex-linked genes – a sex-linked gene has been mapped to a sex chromosome. Linked genes are located close together on a chromosome.
Mapping a trait to a chromosome historically involved classical genetic approaches, including controlled crosses and tracking traits through a pedigree. The beginning of this chapter describes the classical approach to gene mapping from a historical perspective although those techniques have largely been supplanted by tools of molecular genetics.
Since the early 2000s, when genomic sequence data became widely available, genomic comparisons called genetic association studies have more commonly been used to map phenotypes to particular parts of the genome. Genetic association studies can also be used to identify regions of the genome that influence a multifactorial trait.
This module begins with a discussion of landmark studies that allowed researchers to link phenotypes with specific chromosomes using two- and three-point test crosses. It continues with examples of how tools of molecular genetics are used in mapping and ends with modern examples of mapping and a brief discussion of genome-wide association studies.
Media Attributions
- ALS Ice Bucket Challenge © Wikipedia is licensed under a CC BY-SA (Attribution ShareAlike) license
- Stephen Hawking and Lucy Hawking © NASA is licensed under a Public Domain license
- Locke, J. ‘Open Genetics Lectures’ textbook for an Introduction to Molecular Genetics and Heredity (BIOL207). Borealis https://doi.org/10.7939/DVN/XMUPO6 (2017). ↵
- Nickle and Barrette-Ng. Open Online Genetics. in Open Online Genetics (2016). ↵
- Ice bucket challenge: What’s happened since? BBC News (2015). ↵
- Kenna, K. P. et al. NEK1 variants confer susceptibility to amyotrophic lateral sclerosis. Nat. Genet. 48, 1037–1042 (2016). ↵