Multigenic Inheritance
Objectives
- Define the following terms: epistasis, complementation, quantitative trait locus
- Recognize epistasis and complementation in a genetic cross
- Predict offspring ratios from a cross involving epistasis
- Explain how quantitative trait loci contribute additively to a phenotype
- Use a complementation test to determine how many genes are involved in a phenotype
Introduction
In previous chapters, we’ve focused primarily on traits that are controlled by single genes. However, many characters are controlled not by one single gene, but by several genes working together to contribute to the phenotype! Several genes can work together additively to contribute to a phenotype, as in the case of traits like height or skin color. The action of individual genes can also cancel each other out, in some cases.
Because several genes can work in concert to control a single phenotype, the inheritance patterns we see when tracking these types of multigenic traits are different from what was discussed in the unit on Basic Heredity. In this module, we will discuss how epistasis, complementation, and quantitative trait loci affect expected offspring ratios.
The complex and variable coat or feather color in many animals is an example of multigenic inheritance. In dogs, for example, different genes specify hair length (long or short), coat texture, fur color (yellow or non-yellow), Pigment intensity (intense or pale/dilute) pigment, white fur or non-white fur, piebald (white) patches. Alleles for each of these genes can be present in many different combinations, giving many color combinations of dogs.
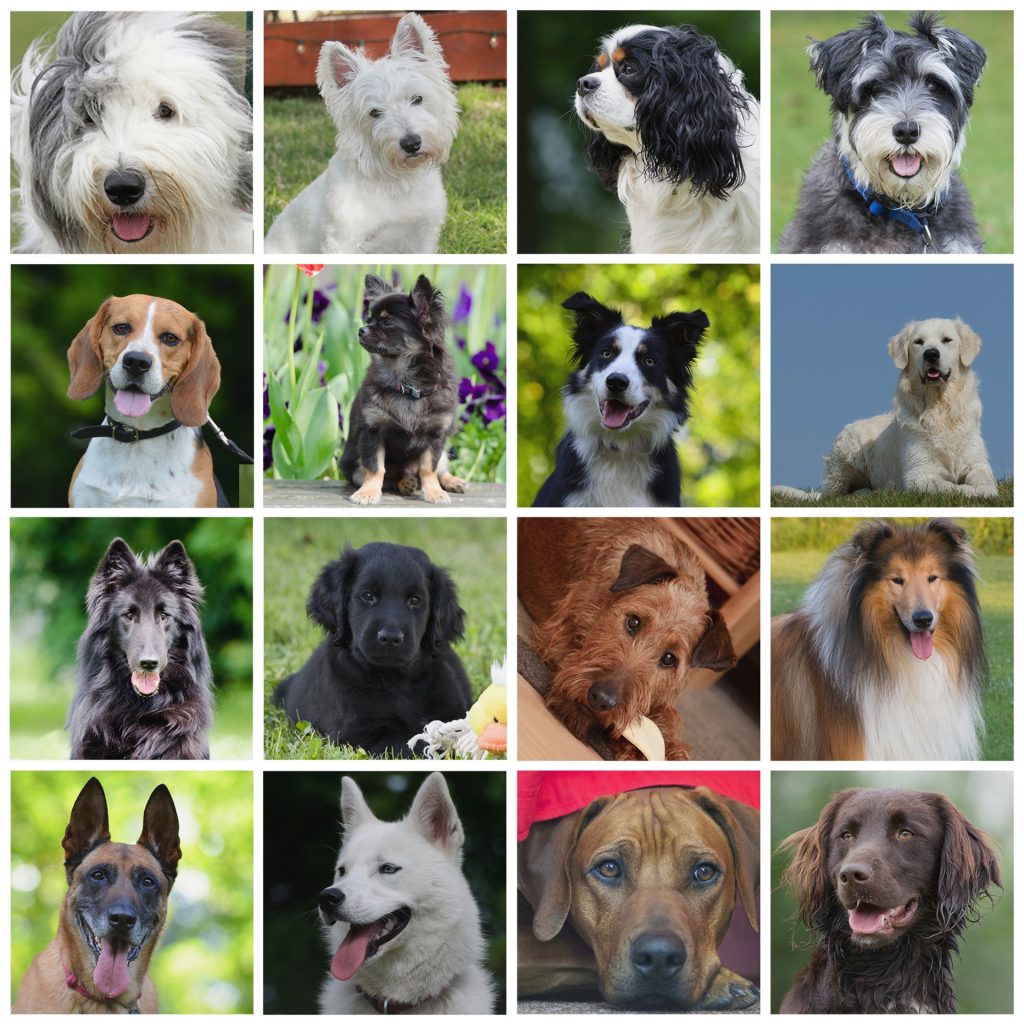
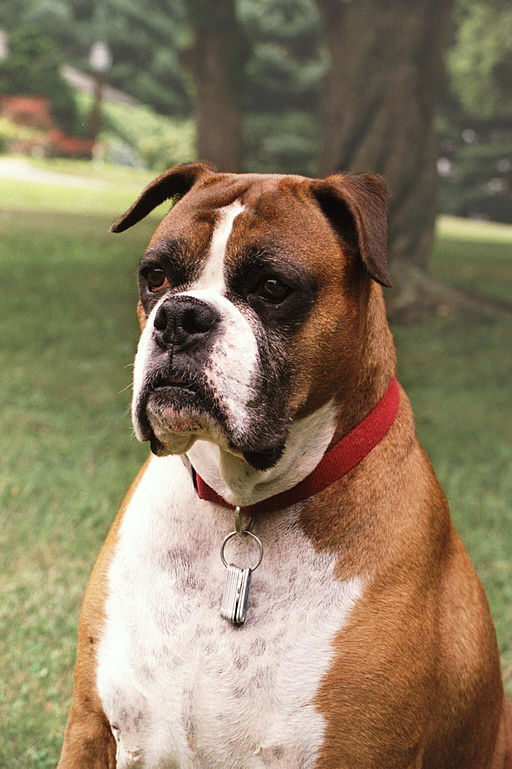
A list of some of the genes involved in controlling coat appearance in dogs is shown in Table 1. Historically, dog breeders came up with single-letter notations to describe the genes they tracked, as they intentionally bred dogs with specific features. For example, a single gene denoted as B seemed to distinguish between black and brown pigments, with Black (B) being dominant and brown (b) being recessive. These concepts of dog genetics were pretty abstract, just like in our unit on Basic Heredity: most dog breeders knew whether their dogs were homozygous black (BB), heterozygous black (Bb), or homozygous brown (bb), based on their parentage and/or the puppies they produced. They tracked traits through generations and counted offspring ratios just like you did in the Basic Heredity unit.
Modern tools of molecular genetics have allowed researchers to identify the part of the dog’s genome that corresponds to those genes. This is called mapping a gene to a chromosome, and it is discussed more in detail in another module. In many cases, researchers found that traits map to genes that they already recognized. The names of those genes and their functions are also shown in Table 1. Why study dogs?
Interestingly, because the dog and human genome are about 95% identical, what we learn from dog genetics is directly applicable to human health. Dogs are a very powerful model organism – an organism that we can study to learn about the underlying principles that govern genetics in many species. Dogs are unique in that they have wide phenotypic variation – a huge Great Dane and a tiny Chihuahua are the same species – but centuries of inbreeding have created specific breeds that are genetically very homogenous. Comparing the genomes of dog breeds with different phenotypes can help identify loci linked with those phenotypes, and comparing the human and dog homologs of the genes can help medical researchers understand how changes in those genes affect human health.
It’s important to recognize that molecular genetics is still a pretty young science – the Human Genome Project was completed in 2003[1], and a dog’s genome was first sequenced in 2005. (Her name was Tasha, and she was a boxer. Her picture is shown in Figure 2.) Almost all the molecular and biochemical information about the genes listed in Table 1 is less than 20 years old. There’s still a lot we don’t know. Check out the Dog Genome Project from Elaine Ostrander’s laboratory at the National Human Genome Research Institute for information about current research into dog genetics.
Feature | Classical genetics notation | Phenotypes | Gene name |
Fur texture | Cu/Cuc | Curly/smooth fur | KRT71 |
Fur color | B/b | Black/Brown fur | TYRP1 |
Fur color | E/e
and other alleles |
Not yellow/Yellow fur
(other alleles change pattern of dark coloration) |
MC1R |
Fur color | D/d | Full color/“dilute” pigment | MLPH |
White patches | S/sp
and other alleles |
No white/ white spots or patches
(an allelic series influences the extent of white) |
MITF |
Fur length | none | Short hair/long hair | FGF5 |
Media Attributions
- Dogs-1024×1024-1 © Karen Arnold is licensed under a Public Domain license
- Genome-Boxer © Nature Education is licensed under a All Rights Reserved license
- First complete sequence of a human genome. National Institutes of Health (NIH)https://www.nih.gov/news-events/nih-research-matters/first-complete-sequence-human-genome (2022). ↵