Three dimensional DNA structure during replication
Prokaryotic chromosomes are circular and typically have one origin of replication. The whole replication process continues in both directions away from the origin until the whole chromosome has been replicated (Figure 10A). The intermediate structures formed by the replication of a circular chromosome are sometimes called theta structures, since they look like the Greek letter theta θ. Another view of the process can be seen in Figure 10B, with an animated version online at Wikimedia Commons.
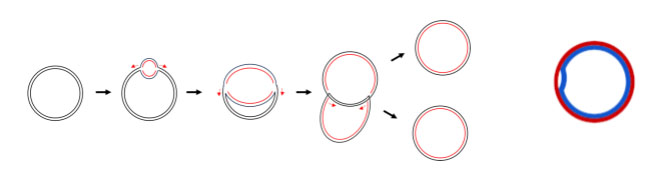
In this description and in the figures, we’ve presented this as if the leading strand is synthesized first and the lagging strand second. Even the names give this impression! But the synthesis of leading and lagging strands happens simultaneously. This requires the proteins involved in this process to work in concert. While the leading strand polymerase acts continuously on the leading strand template, the lagging strand polymerase dissociates after each Okazaki fragment, rebinding to each new primer. Throughout this process, the two polymerases stay linked so that as the replication fork moves away from the origin, both strands are replicated at once. To accomplish this, all of the replication participants must be organized very specifically in three dimensional space.
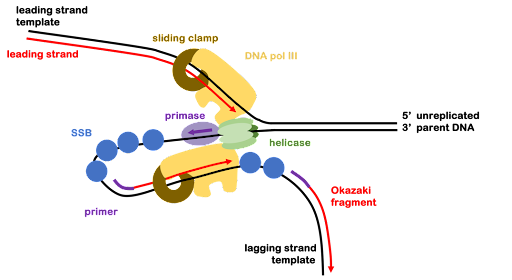
As the replication fork opens, the lagging strand template becomes looped around, as shown in Figure 11, where the lagging strand has been folded under itself to bring the two polymerases closer together. This is called the trombone model of replication because as the fork progresses away from the origin, this loop appears to grow and shrink as the DNA template moves in relation to the polymerase. The entire process is shown in this animation of the replication process, produced by HHMI Biointeractive.
Note: Although they are not shown in the figures of this text, additional subunits of the replisome also participate in the replication process. Additional components of the replication machinery help perform functions like loading and unloading the clamp for new Okazaki fragments and keeping the leading and lagging polymerases together so that both template strands are replicated in concert.
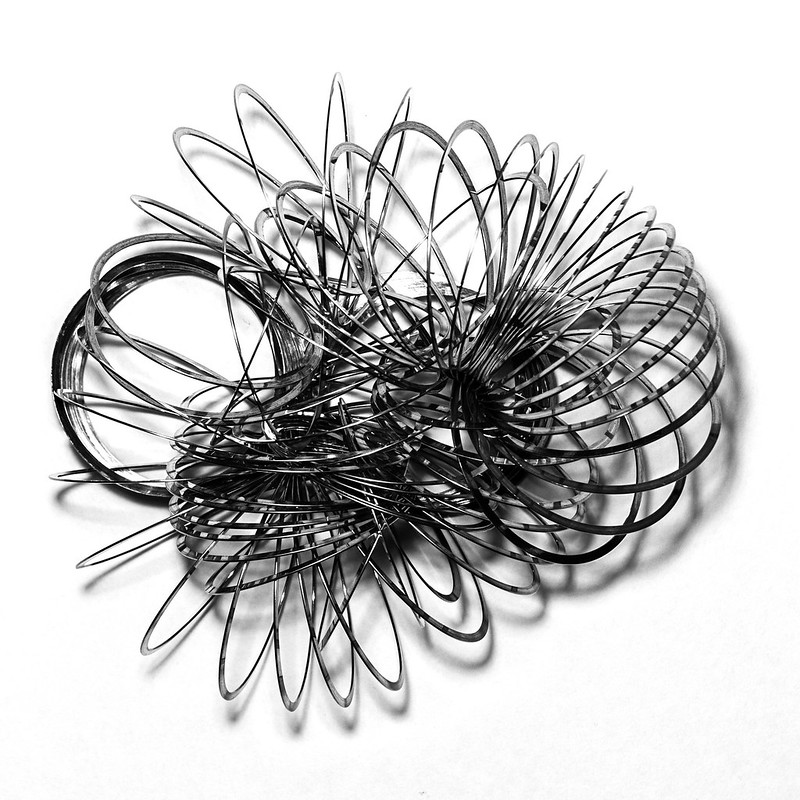
The three-dimensional structure of the replisome and the length of the DNA strands also cause certain difficulties. Have you ever tried to untangle a spring toy (Figure 12), comb through long tangled hair, or wrestle with the power cord on a hand-held appliance? Just like those examples, the parent and daughter DNA strands become twisted around one another in a way that makes it difficult to both melt the template DNA and separate the two daughter duplexes after replication is complete.
A class of enzymes called topoisomerases relieve the torsional strain caused by melting the double helix and untangle the daughter DNA.
Media Attributions
- Bacterial chromosomes © Catherinea228 via. Wikipedia is licensed under a CC BY-SA (Attribution ShareAlike) license
- Trombone model © Amanda Simons is licensed under a CC BY-SA (Attribution ShareAlike) license
- Tangled spring toy © Val D'Aquila via. Flickr is licensed under a CC BY-SA (Attribution ShareAlike) license