Mechanisms of evolution
In addition to mutation, genetic drift, gene flow, natural selection, and assortative mating are factors that influence the evolution of a population.
Natural selection
Natural selection is probably what many people think of as driving evolution. This is Charles Darwin’s proposal for “survival of the fittest”, where “fitness” is determined by the likelihood of reproducing. Selection results when one variant confers some sort of advantage over another. An individual with the advantageous variant is more likely to reproduce, contributing the advantageous allele to the gene pool of the next generation (Figure 3). An individual with the disadvantageous variant is less likely to reproduce, so the disadvantageous allele is likely to make up a smaller percentage of the gene pool.
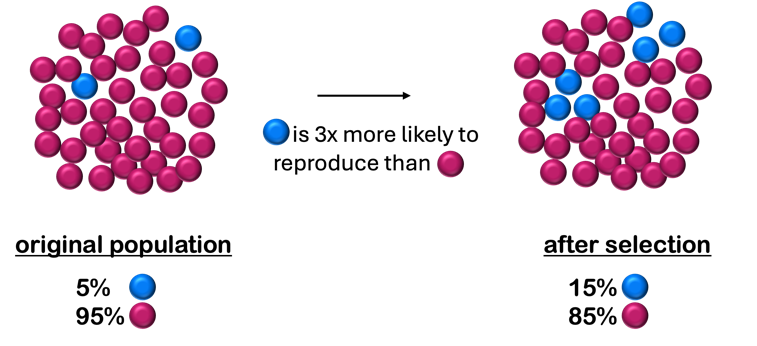
On a molecular level, this is the reason why certain sequences in the genome are more conserved than others. For example, the function of many of the consensus sequences we described in the molecular genetics modules depend on DNA: protein interactions. Any changes to those sequences would reduce function and presumably negatively impact the fitness of the organism. So, any individual who acquires a mutation in a consensus sequence may be less likely to reproduce. Such conserved regions of the genome are typically under great selective pressure against mutation.
Note that in populations of diploid organisms, it is not always a homozygote that will have an advantage! This, called the heterozygote advantage, is discussed further in the chapter on Population Genetics. Heterozygote advantage can result in the maintenance of otherwise deleterious alleles in a population.
Note also that if environmental conditions change, the selective pressure on a population can change, too. For example, the introduction of a new pathogen might selectively affect individuals with some variants rather than others. Or an increase in environmental temperature might favor some variants vs others. Because of this, genetically diverse populations tend to adapt better to changing conditions than populations that are genetically homogenous.
Genetic drift
While natural selection is a directional change due to some sort of pressure, genetic drift refers to the random variations that happen from generation to generation. In any population, not all individuals will reproduce at equal levels. Which individuals reproduce can affect the frequency of alleles in a population. Over many generations, genetic drift can change a population, illustrated in Figure 4.
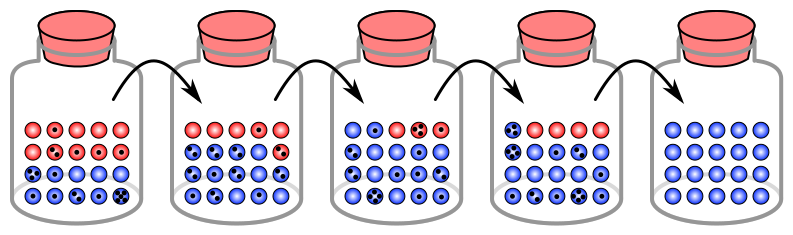
Genetic drift tends to be amplified in small populations where small random fluctuations can have a big effect. To use an analogy: you’d expect a coin flip to generate heads about 50% of the time. But if you flip a coin 4 times, you won’t always get 2 heads and 2 tails. Sometimes, you might get 3 heads/1 tail (75% heads). And sometimes you might even get all heads (100%)! On the other hand, if you were to flip a coin 1,000,000 times, you might not have exactly 50% heads, but you would not expect 1,000,000 heads in a row.
Two extreme examples of genetic drift magnified by a change in population size should be mentioned: the founder effect and the bottleneck effect.
The founder effect happens when a small fraction of a population founds, or begins, a new population, as shown in Figure 5. The smaller population can have a different genetic makeup than the original population. For example, if a rare allele is present among the founders, that allele may be more common in the new population. If the populations remain apart for generations, additional drift can further separate the populations.
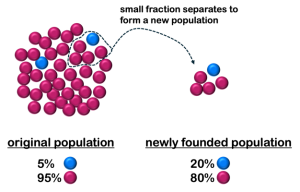
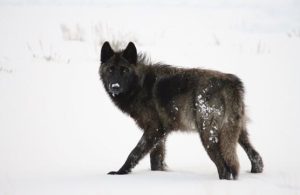
An example of the founder effect is seen in the wolves of Yellowstone National Park (Figure 6). Hundreds of years ago, grey wolves were found across the northern and western United States. But in the past 150 years, wolves vanished across most of the United States, mostly due to hunting. This had profound consequences on the ecosystem. In 1995, biologists reintroduced a small population of wolves to locations in Yellowstone National Park, in the US states of Wyoming and Montana. The wolves of Yellowstone have been monitored ever since.
Even though it is called a grey wolf, the animal naturally varies in coat color. In most North American wolf populations, less than 5% of wolves are black. The Yellowstone population is about 50% black, reflecting the gene pool of the small number of wolves reintroduced to the park[1].
The bottleneck effect occurs when a natural disaster decimates a large percentage of a population. The resulting population may have different allele frequencies from the parental population, as shown in Figure 7. In contrast to an event of natural selection, in which one variant has an advantage over another, a bottleneck event kills off individuals randomly. Bottleneck events typically reduce the genetic diversity of a population. Many endangered species have reduced genetic diversity (due to bottlenecks caused by overhunting or overfishing).
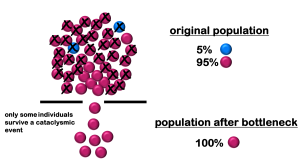
Gene flow
Gene flow results from the migration of individuals (and genes) from one previously isolated population to another. It is also called admixture. Admixture can keep somewhat isolated populations of a species from diverging much from one another. This can happen between populations of the same species or different species. Interspecies gene flow is called horizontal gene transfer.
Analysis of the human genome reveals that many people have Neanderthal DNA in their genomes, offering evidence that ancient Homo sapiens interbred with Homo neanderthalensis. This is an example of gene flow between two populations of hominins.
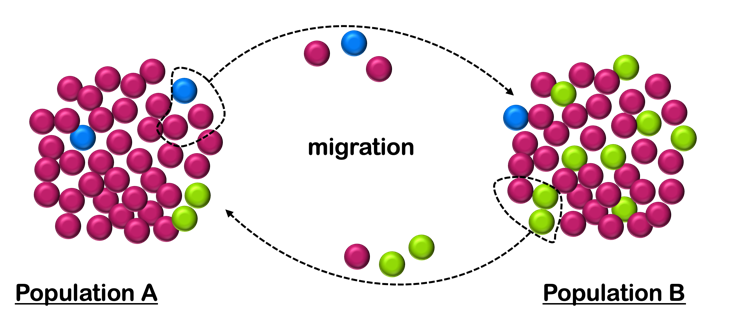
Assortative mating
Assortative mating can also influence evolution in a population. Most studies of populations assume that mating occurs randomly within a geographical population, but that’s not always the case. In human populations, for example, choice of partner greatly depends on social and cultural influences, as well as geography. And even in animal populations, selection of a mate can be nonrandom. For example, several studies show that butterflies have a preference to choose a mate with similar color patterns[2].
If individuals preferentially select mates like themselves, inbreeding can further amplify rare traits in a population. Discussed briefly with consanguineous pairings in the Pedigree chapter, inbreeding means that individuals with shared genetics produce offspring. Every individual in a population is likely to have a few rare, recessive variants. Most of the time, the rare recessive traits will not manifest in offspring since it would be unlikely to have two parents both share the same rare variant. But with inbreeding that becomes more likely.
The selective breeding that gave rise to many dog breeds is an example of this. Dog breeds tend to be genetically homogenous, specifically bred for desired characteristics. As a result, many dog breeds have an increased incidence of genetic disease: Dalmatians are prone to deafness, German Shepherds are prone to hip dysplasia, and Doberman Pinchers are prone to hypothyroidism, among others.
H5P Test your understanding
Media Attributions
- Figure 3 Natural Selection © Amanda Simons is licensed under a CC BY-SA (Attribution ShareAlike) license
- Figure 4 Genetic Drift © Based on File Random_sampling_genetic_drift.gif is licensed under a CC BY-SA (Attribution ShareAlike) license
- Figure 5 Founder Effect © Amanda Simons is licensed under a CC BY-SA (Attribution ShareAlike) license
- Figure 6 Black Wolf is licensed under a Public Domain license
- Figure 7 Bottleneck effect © Amanda Simons is licensed under a CC BY-SA (Attribution ShareAlike) license
- Figure 8 Gene Flow © Amanda Simons is licensed under a CC BY-SA (Attribution ShareAlike) license
- Park, M. A. P. B. 168 Y. N. & Us, W. 82190-0168 P. 307-344-7381 C. Gray Wolf - Yellowstone National Park (U.S. National Park Service). https://www.nps.gov/yell/learn/nature/wolves.htm. ↵
- Butterflies are genetically wired to choose a mate that looks just like them. ScienceDaily https://www.sciencedaily.com/releases/2019/02/190207142148.htm. ↵