Mendel’s Second Law
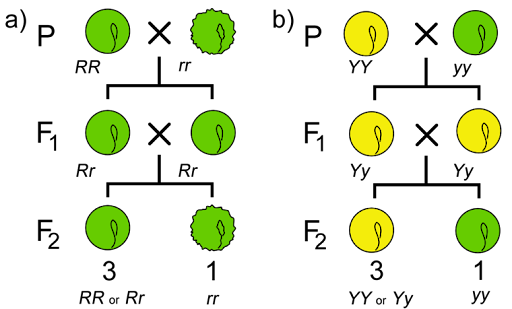
Mendel’s second law addresses the inheritance of alleles of multiple genes.
Mendel’s initial experiments with pea plants looked at what happens when plants that vary in one characteristic – for example, crossing plants with yellow seed and green seeds. He followed this up with experiments where plants varied in multiple characteristics – for example, crossing plants with round, yellow seeds and wrinkled, green seeds. He wanted to know if traits would segregate independently or whether traits that were coupled in the parents would be inherited as a single unit.
Mendel crossed peas that varied in two traits: seed color and seed shape. He crossed a true-breeding plant that had yellow and round seeds with a true-breeding plant that had green and wrinkled seeds. All the F1 offspring were Yellow and Round. The F1 offspring were dihybrids, meaning they were heterozygous at two genes (genotype YyRr). Mendel then self-crossed the F1. The F2 offspring could have any combination of traits: Yellow and round or green and wrinkled like the parents, or a recombinant phenotype of yellow, wrinkled or green, round.
For example, if he crossed parental peas with Yellow and Round seeds x green and wrinkled seeds, offspring could have any combination of traits: Yellow and round or green and wrinkled like the parents, or a recombinant phenotype of yellow, wrinkled or green, round.
He found that traits that were present together in the parents were not necessarily inherited together in the offspring. This led him to the Law of Independent Assortment.
Law of Independent Assortment: The distribution of alleles from one gene to offspring is not dependent on the distribution of alleles from another gene.
Or, in other words, when gametes are produced, the alleles from each gene are passed to the next generation independently of others.
Recall that in Mendel’s first set of experiments, there was a 3:1 ratio of dominant: recessive traits from a monohybrid cross (Aa x Aa). This is shown in Figure 6. Also recall: a monohybrid cross indicates that we are tracking the inheritance of one gene, but the organism itself still has tens of thousands of other genes that we are not paying attention to.
In a dihybrid cross (AaBb x AaBb), the 3:1 ratio still holds true for each of the genes. Yellow, Round seeds crossed with green, wrinkled seeds will give an F1 dihybrid with the Yellow and Round phenotypes. When the F1 dihybrid is self-crossed, ¾ of the offspring are Yellow, and ¼ of the offspring are green. ¾ of the offspring are Round, and ¼ are wrinkled. But how many of the Yellow seeds are also Round? How many of the Yellow seeds are wrinkled?
Mendel’s results showed a 9:3:3:1 ratio of Yellow, Round: Yellow, wrinkled: green, Round: green, wrinkled. Or, in other words:
- 9/16 of the offspring have both dominant traits (Yellow and Round)
- 3/16 of the offspring have one dominant and one recessive trait (Yellow and wrinkled)
- 3/16 of the offspring have one recessive and one dominant trait (green and Round)
- 3/16 of the offspring have both recessive traits (green and wrinkled)
This is illustrated in Figure 7.
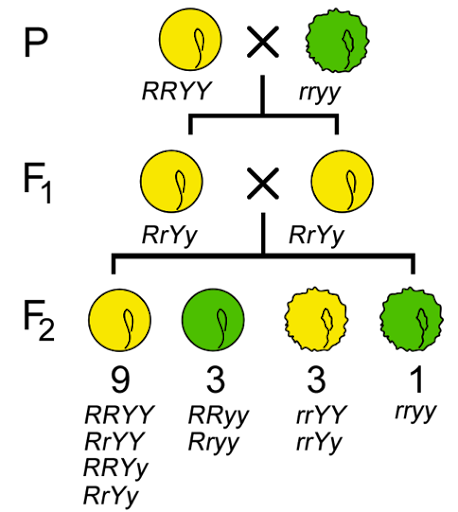
These observed numbers are consistent with the probability of two independent events occurring together.
The multiplication rule of probability allows us to calculate the probability of two independent events both occurring, just by multiplying the two independent probabilities together. For example, if the round and the yellow genes are inherited independently, the probability of obtaining offspring that are both round and wrinkled is the probability of round offspring multiplied by the probability of wrinkled offspring. This is illustrated in the equation below, where P stands for Probability.
Pround and yellow = Pround * Pyellow
Using the multiplication rule:
In a monohybrid cross for seed color, we would expect ¾ of the progeny were yellow. In a monohybrid cross for seed shape, we would expect ¾ of the progeny were round. Therefore, for a dihybrid cross for seed color and shape, ¾ round × ¾ wrinkled = 9/16 of the progeny would be both yellow and round. Likewise, ¾ × ¼ = 3/16 of the progeny would be both round and green and so on. The calculations for all four potential combinations of traits are shown in Table 1.
Phenotypic class | Probability of seed color | Probability of seed shape | Probability of combined phenotype |
---|---|---|---|
Yellow and Round | ¾ | ¾ | ¾ * ¾ = 9/16 |
Yellow and wrinkled | ¾ | ¼ | ¾ * ¼ = 3/16 |
green and Round | ¼ | ¾ | ¼ * ¾ = 3/16 |
green and wrinkled | ¼ | ¼ | ¼ * ¼ = 1/16 |
The multiplication rule of probability thus predicts a 9:3:3:1 ratio of phenotypic classes for a dihybrid cross if the alleles are indeed inherited independently. Mendel observed close to a 9:3:3:1 ratio for all combinations of traits he reported.
The Law of Independent Assortment means that we can treat genes independently, even if we are tracking more than one trait at a time.
Exceptions to the Law of Independent Assortment
Mendel did have a bit of luck: all of the traits he chose are encoded on different chromosomes in the pea genome. Since Mendel’s time, it has become clear that genes located close together on the same chromosomes (so-called linked genes) do not follow the law of independent assortment. These exceptions to the rule will be discussed in the module on Linkage.
Media Attributions
- Monohybrid crosses © Nickle and Barrette-Ng via. Open Online Genetics is licensed under a CC BY-NC-SA (Attribution NonCommercial ShareAlike) license
- Pure breeding © Nickle and Barrette-Ng via. Open Online Genetics is licensed under a CC BY-SA (Attribution ShareAlike) license