One promoter can be regulated by both activators and repressors: The lac operon
One of the first- and best-studied systems of gene regulation is the lac operon in E. coli. In a series of experiments conducted in the late 1950’s, Francois Jacob, Jacques Monod, and Arthur Pardee collaborated to determine how E coli lactose-metabolizing enzymes are regulated. These experiments are called the PaJaMa (or sometimes PaJaMo) experiments, a name created from the first letters of the researchers’ last names. The specifics of the PaJaMa experiments are discussed later in this chapter.
E coli can use many different sugars as a food source although glucose is usually the most efficient option if it is available. Other sugars like lactose can be used as an energy source, but additional enzymes are needed for their metabolism. Lactose is a disaccharide comprising glucose and galactose monosaccharides (Figure 9).
In E. coli, metabolism of lactose begins with two proteins: lac permease, which is a transporter that brings lactose into the cell, and β-galactosidase, which breaks the glycosidic bond connecting the monosaccharides, producing glucose and galactose. Lac permease is encoded by the lacY gene, and β-galactosidase is encoded by the lacZ gene.
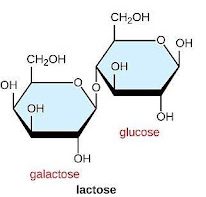
The metabolism of lactose requires the proteins to work together, so the genes must be co-regulated. LacZ, lacY, and a third gene, lacA, are arranged in an operon. LacA encodes trans-acetylase, the role of which isn’t clear in lactose metabolism.
The gene of the lac operon are grouped together under the control of the lac promoter, called lacP (Figure 10). The -10 and -35 sequences of the lac promoter are not a perfect match to consensus, so lacP is a weak promoter.

Transcribing and translating these genes takes up a lot of energy, so these genes are only expressed when they are needed: i.e. when lactose is available but the preferred energy source, glucose, is not. To accomplish this control, transcription is regulated by two transcription factors: the lac repressor (encoded by the gene lacI, separate from the operon), and catabolite activator protein, or CAP, which is not shown in this image.
LacI is a lactose sensor: LacI blocks transcription if there’s no lactose
The lac repressor, as the name suggests, represses expression – it turns the operon “off”unless lactose is present. It is a lactose sensor. The lac repressor protein binds to sites called operators, which surround the promoter and overlap with the +1 site of the operon. The operators are labeled “O” in Figure 10. When there is no lactose present, the repressor binds to the operators, blocking transcription. When there is lactose present, the repressor releases the operators and transcription can occur. This is shown in Figure 11.
Note that the repressor acts as both a dimer and a tetramer: two repressor polypeptides bind to each operator, and the operator-bound dimers can come together to form a tetramer that further blocks access of RNA polymerase to the promoter.
Be careful not to confuse the terms operon and operator! An operon is a single transcriptional unit that includes multiple genes, while the operator is a DNA element that is recognized by a repressor.
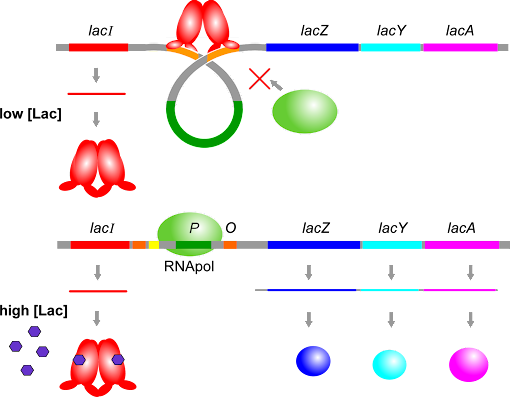
Test Your Understanding
CAP is a glucose sensor: CAP activates transcription only if there’s no glucose
The lac operon is not expressed at high levels when glucose is present, via a mechanism called catabolite inhibition. But this is not negative regulation! Instead, the low expression is actually the absence of a positive regulator.
The lac operon can be negatively regulated (or turned off) by the action of the lac repressor. But the absence of the repressor is not enough to produce great numbers of lac mRNA. This is because the lac promoter is not a very good match to the consensus -10 and -35 sequences. In fact, the weak promoter shown back in Figure 3 is actually the sequence of the lac promoter. The sigma factor and the RNA polymerase bind inefficiently to the imperfect sequence, which in turn leads to inefficient transcription of the operon.
In order to produce high amounts of the lac RNA, the operon needs an activator, CAP. CAP stands for catabolite activator protein. CAP binds to the CAP binding site (CBS in Figure 10) upstream of the -35 box. CAP also binds to RNA polymerase, stabilizing it on the promoter and increasing transcription.
Like the lac repressor, CAP is an allosteric protein that exists in both active and inactive conformations. CAP is not always active: CAP can only bind to DNA when it is complexed with cyclic AMP (cAMP). For this reason, CAP also sometimes goes by the name cAMP receptor protein, or CRP for short.
cAMP levels are high typically only when there is not much glucose in the environment, because low levels of glucose trigger an enzyme called adenylate cyclase to produce cAMP from ATP, as shown in Figure 12. So CAP only binds to the CBS to activate the lac operon when glucose is low. High levels of glucose inhibit the action of adenylate cyclase, inactivating CAP, so the operon will not be activated.
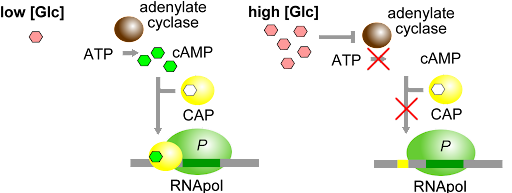
Test Your Understanding
CAP and the lac repressor work together to react to changing conditions
Remember, glucose is an optimal sugar source for E.coli, but lactose is acceptable if that’s what is available. The lac repressor and CAP allow the cell to react to changing environmental conditions. Lac essentially acts as a lactose sensor, and CAP essentially acts as a glucose sensor, although neither factor binds directly to the sugar. The cell can therefore react to four separate combinations of environmental conditions: with glucose alone, with both glucose and lactose, with lactose alone, and neither.
When glucose alone is present, the repressor is bound to the operator and CAP is absent. This leads to no transcription.
When both glucose and lactose are present, the repressor is not bound, but neither is CAP. This leads to low (basal) levels of transcription since the operon is neither repressed nor activated.
When lactose alone is present, the repressor is not bound, and CAP is! This leads to high levels of transcription.
And when neither sugar is present, both the repressor and activator can bind. Who wins? The repressor wins: the operon is not transcribed. This is because of the way the activator and repressor function: with the repressor bound to the operator, there is no room for the polymerase to bind, even with the activator to assist. Two things cannot be in the same place at the same time.
This is summarized in the flow chart in Figure 13.
Sugars present | Is the repressor bound? | Is CAP active? | Expression |
Glucose | Yes | No | None |
Glucose + Lactose | No | No | Basal (low) |
Lactose | No | Yes | High |
Neither glucose nor lactose | Yes | Yes
(but doesn’t matter, since repressor blocks transcription) |
None |
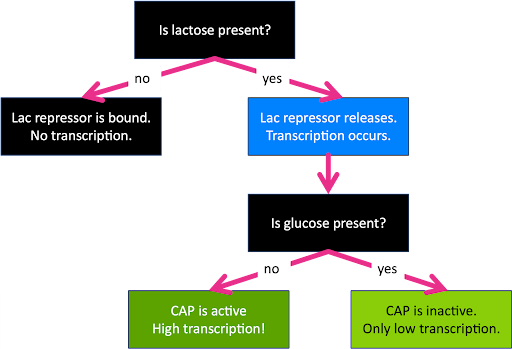
Test Your Understanding
Lac repressor mechanism of action
The lac repressor is a great example of the modular nature of many transcription factors: the protein has separate domains that perform different tasks. These can be seen in Figure 14. If you would like to explore a 3D animated version of this structure that you can drag and turn, you can find one at Proteopedia.
At the top of Figure 14 you can see the DNA binding domain of the protein, bound to operator DNA (in yellow). The repressor binds as a dimer to the operator consensus sequence 5′TGGAATTGTGAGCGGATAACAATT3′[1]. One operator is located between the -10 and +1 sites of the gene, and the other is farther upstream, as shown in Figure 11. When the repressor binds to the operator, the repressor takes up enough physical space that there is no room for sigma/polymerase to bind: the bound repressor blocks transcription, just like the repressor example in Figure 4.
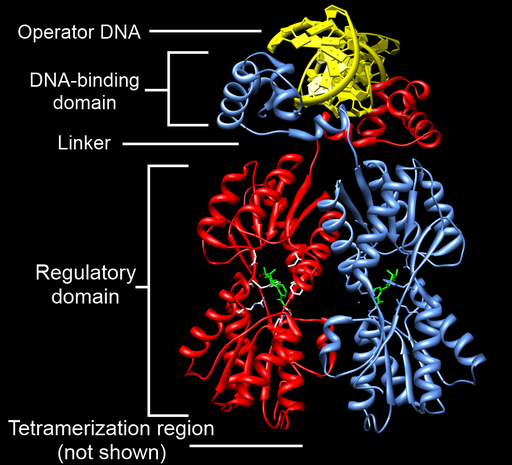
Although the lac repressor acts as a lactose sensor, it does so indirectly. The repressor only binds to the operator when there is no lactose present. When there is lactose present, a metabolite of lactose called allolactose binds to the repressor via the regulatory domain of the protein. The allolactose-bound repressor changes conformation and can no longer bind to the operator. This frees up the promoter, making transcription of the lac operon possible. Because the repressor exists in multiple conformations, it is called an allosteric protein.
The presence of allolactose leads to increased expression of the operon, so allolactose is said to be an inducer of the operon. Note that other molecules can also induce the operon. For example, a molecule called IPTG is often used as an inducer of the lac promoter in a laboratory setting.
Although it is not shown in Figure 14, the repressor has an additional domain that allows two DNA-bound dimers to come together to form a tetramer. This allows the repressors on both operators to interact, forming a loop of the intervening sequence between the two operators. This larger complex likely further inhibits transcription. Figure 15 shows the tetramerization between two DNA-bound dimers.
This modular nature of the repressor is characteristic of other transcription factors as well. It is common for a transcription factor to have separate DNA binding domains and regulatory domains.

Genetic mutations of the lac operon
Experiments like the PaJaMa experiments allowed geneticists to understand the interactions between DNA elements and protein factors. Such experiments typically rely on a collection of bacteria that have mutations in one or more of the components of the lac operon:
- Null mutations in the structural genes, called Z– and Y–. These mutations cannot produce β-galactosidase or lac permease depending on the mutation. (Null means “none” or “zero”.)
- Constitutive mutation of the operator, Oc. These mutations are in the operator sequence that prevents the repressor from binding so that the proteins of the operon are always produced, regardless of environmental conditions. (The word constitutive means “always on”.)
- Loss of function mutation of the lac repressor, called I–. These may be null mutations with no protein produced, or they may have a mutation in the DNA binding domain of the repressor so that it cannot bind to the operator. These mutations, like the Oc, prevent the operon from being turned off, so it is also considered a constitutive mutation.
- Gain of function mutations in the lac repressor, called IS. These so-called “super repressors” have a mutation in the allolactose binding domain, so that sugar-sensing domain does not function. These super repressors never release the operator, so the operon is never expressed.
These mutants are compared to wild-type versions of all genes, usually indicated with a superscript “+”: I+, O+. Z+, Y+.
These experiments relied on the use of partial diploid bacteria: bacteria that carried an extra copy of the lac operon on an extrachromosomal piece of DNA called the F’ episome.
F’ is also called the fertility factor: copies of F’ are transferred between bacteria via conjugation, or bacterial mating. For more information on conjugation, see this video from HHMI Biointeractive.
In this case, the extra copy of the lac operon allowed researchers to determine which genetic elements act in cis (that is, only upon the chromosome on which they reside) or trans (upon other chromosomes, too). Those mutations that act in cis are the mutations in DNA elements – loss of function in a promoter or operator, for example, affects transcription of adjacent genes, but has no effect on the transcription of genes on another chromosome. On the other hand, mutations that act in trans are mutations in a protein factor, having the potential to affect the transcription of both copies of the lac operon.
The extra copy of the chromosome also allowed these bacterial geneticists to determine whether a mutation was dominant or recessive. For example, the gain-of-function super repressor IS is a dominant, trans-acting mutation: even if a second normally functioning repressor is present, the super-repressor can bind to both operators and repress transcription of both copies of the operon. The constitutive operator OC is a dominant cis-acting mutation: it only affects the expression of adjacent genes, but the always-on expression of one copy of the operon means that the cell always has β-galactosidase and permease, regardless of whether the second copy of the operon is properly regulated.
Test Your Understanding
Media Attributions
- Structure of lactose © CNX OpenStax is licensed under a CC BY-SA (Attribution ShareAlike) license
- E. coli chromosome © Online Open Genetics (Nickle and Barrett-Ng) is licensed under a CC BY-NC-SA (Attribution NonCommercial ShareAlike) license
- Lactose is not present © Original-Deyholos-CC:AN, via Online Open Genetics. is licensed under a CC BY-NC-SA (Attribution NonCommercial ShareAlike) license
- CAP bound to cAMP © Nickle and Barrett-Ng via. Online Open Genetics ().
- Lac operon flow chart © Amanda Simons is licensed under a CC BY-SA (Attribution ShareAlike) license
- LacI Dimer Structure © SocratesJedi at English Wikipedia is licensed under a CC BY-NC-SA (Attribution NonCommercial ShareAlike) license
- Bound Tetrameric Lac Repressor © SocratesJedi at English Wikipedia is licensed under a CC BY-NC-SA (Attribution NonCommercial ShareAlike) license
- Gilbert, W. & Maxam, A. The Nucleotide Sequence of the lac Operator. Proc. Natl. Acad. Sci. U. S. A. 70, 3581–3584 (1973). ↵