One transcription factor can be both an activator and repressor: The lambda phage life cycle
A second example of prokaryotic gene regulation is the switch between lytic and lysogenic growth in the bacteriophage λ (lambda). Bacteriophages, or phages for short, are viruses that infect bacteria: yes, even bacteria can catch a virus!
The structure of phage λ is shown in Figure 16. The left and center image show an electron micrograph and artist’s interpretation, respectively. On the right in Figure 16 is a cartoon of basic phage structure. Every phage has a “head” structure, which encases the genome. A phage also has a long sheath and a base plate with tail fibers extending out and down. The tail fibers help the phage attack and attach to a target bacterium. After attachment, the phage will inject its DNA through the shaft into the host cell.
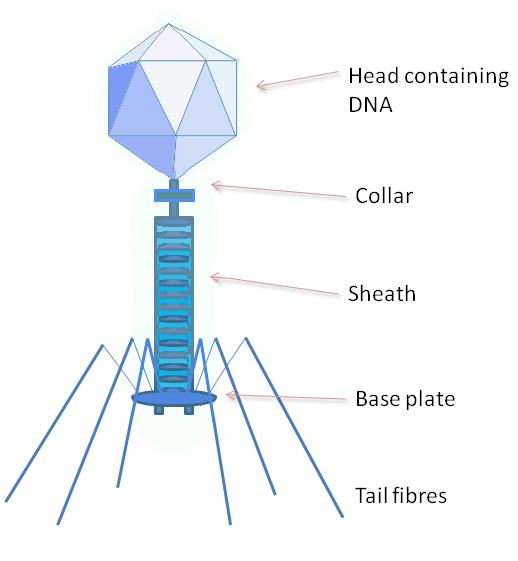

After the viral genome enters the host cell, the bacteriophage may switch between either lytic or lysogenic growth. During lytic growth, shown on the top in Figure 17, the phage genome is replicated, transcribed, and translated by the host cell, which has been commandeered by the virus to make more viruses. Once new virus particles have been assembled, the cell is lysed, or broken open, releasing the new viral particles to infect other cells.
During lysogenic growth, shown on the bottom in Figure 17, the viral genome is injected into the cell and integrated into the host genome. The integrated viral DNA sequence is called the prophage. It is replicated and passed to daughter cells along with the host genome as the cell divides. The prophage can persist in this fashion indefinitely, but conditions of stress in the host cell can switch the phage to lytic growth.
The switch between lysogenic and lytic growth is controlled by expression three promoters in the phage genome, called PL, PR and PRM, diagrammed in Figure 18A. PL and PR drive expression of genes needed for early stages of lytic growth, and PRM drives expression of the lambda repressor, λCI. The promoters are oriented so they use different strands as template for transcription. In Figure 18, PR uses the bottom strand as template and transcribes to the right in this image. PRM and PR use the top strand as template and transcribe to the left in this image.

λCI is called the lambda repressor, but this is not an accurate name: λCI can act as both a repressor and an activator!
Like the lac repressor, λCI has multiple domains: the N-terminal domain binds DNA. The N-terminal domain also has a patch on the surface that interacts with RNA polymerase. The C-terminal domain is a multimerization domain that allows each molecule of λC to interact with others. λCI functions as a dimer, but each dimer can interact with other dimers to form tetramers or octamers. You can drag, spin, and magnify the structure of λCI at Proteopedia.
λCI binds to operators near these three promoters, but it does not bind equally well to all of them. Dimers of λCI bind best to OL1 and OR1, and this helps additional dimers bind to OL2 and OR2 in what is called cooperative binding. This binding blocks polymerase access to PL and PR and blocks transcription of lytic genes.
But wait, there is more! Although it is called the lambda repressor, the protein can also act as an activator for its own promoter. Part of the lambda repressor binds to RNA polymerase, recruiting it (helping it to bind) to PRM. The lambda repressor therefore acts as its own activator. This is positive autoregulation, where “auto” means it regulates its own gene. This is shown in Figure 18B.
But there is even more! λCI must be carefully regulated so there is enough protein to repress the lytic genes, but not so much that the phage can’t undo this repression when needed. So λCI can act as its own repressor, too! At high concentrations of λCI, OL3 and OR3 will also be occupied by dimers of λCI. This leads to looping of the DNA as shown in Figure 18C, with λCI holding the loop in place and repressing transcription from PRM, too. This is negative autoregulation.
Stress response in the host cell can trigger the switch to lytic growth and release of new phage particles. This happens through cleavage of the λCI protein, which de-represses PR and PL and leads to the production of lytic proteins. One protein produced from PR is called cro. Cro binds to OR3 and blocks transcription of the cI gene. In this way, either lytic genes or lysogenic genes are transcribed, but not both at the same time[1].
You can also see an animated GIF of the switch in action via Google Drive.
So why does the λCI sometimes act as a repressor and sometimes an activator? It has to do with the geometry of binding. OR1 and OR2 are positioned over the -10 and -35 boxes for PR. Remember, repressors can repress just by taking up space around a promoter. Two things cannot be in the same place at the same time, so if λCI is bound then RNA polymerase cannot. On the other hand, when λCI is positioned in OR2 the RNA polymerase-interacting domain is positioned perfectly to stabilize a polymerase bound to PRM. λCI can only activate transcription if it is properly aligned with the promoter.
Test Your Understanding
Media Attributions
- Electron micrograph of Lambda © Photographer: Hans-Wolfgang Ackermann via. Wikipedia is licensed under a CC BY-SA (Attribution ShareAlike) license
- Molecular data of Lambda © Dr. Victor Padilla-Sanchez, PhD via. Wikipedia is licensed under a CC BY-SA (Attribution ShareAlike) license
- Phage structure, © GrahamColm at English Wikipedia is licensed under a CC BY-SA (Attribution ShareAlike) license
- Lytic vs lysogenic © LibreTexts is licensed under a CC BY-NC-SA (Attribution NonCommercial ShareAlike) license
- Lambda repressor © Amanda Simons is licensed under a CC BY-SA (Attribution ShareAlike) license
- Hochschild, A. & Lewis, M. The bacteriophage λ CI protein finds an asymmetric solution. Curr. Opin. Struct. Biol. 19, 79–86 (2009). ↵