Regulation of Gene Expression
Objectives
After completing this module, you should be able to:
- Describe levels of gene regulation.
- Describe how activators and repressors can act to increase or decrease transcription of a gene.
- Explain how activators and repressors can work together to regulate gene expression in response to changing conditions, using the lac operon and bacteriophage lambda as an example.
- Explain how translation of the trp leader results in transcriptional termination.
- Predict how mutations in the lac operon would affect its expression.
- Compare and contrast mechanisms of gene regulation in prokaryotes and eukaryotes.
- Define: gene expression, gene regulation, transcriptome, proteome, activator, repressor, positive regulation, negative regulation, constitutive mutation, attenuation
Introduction to gene regulation
In the chapters on replication, transcription, and translation, we looked at the process by which a cell uses the information stored in the genome to produce RNA and protein. But the process of RNA and protein production is tightly controlled, so only some genes are expressed, or active, at any time.
The genome is the collection of DNA that is present in a cell or organism. Likewise, the transcriptome is the collection of RNA present in a cell or organism. The transcriptome includes protein-coding mRNAs as well as functional non-coding RNAs. The transcriptome can also include multiple forms of an RNA for each gene: edited and alternatively spliced RNA increases the diversity of the transcriptome. The proteome is the collection of proteins in a cell or organism. Just like for RNA in the transcriptome, one gene can contribute many proteins to the proteome, since multiple protein molecules can be generated through edited or spliced RNA molecules. The proteome also includes proteins modified post-translationally, through covalent linkage of small molecules like phosphates, acetyl groups, sugars, or lipids.
This is illustrated in Figure 1.
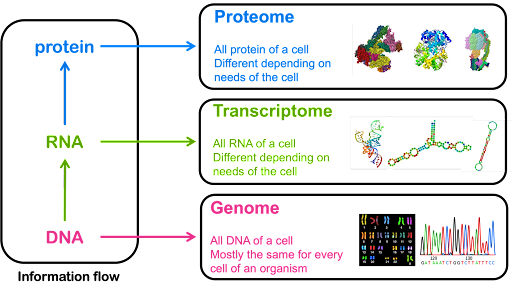
Although the genome is mostly the same in every cell of a multicellular organism, this is not true of the transcriptome and proteome. For many (but not all) genes, the ultimate gene products are protein molecules. Different proteins are needed for different cellular functions, but not all proteins are always needed by all cells. It’s inefficient – and, sometimes, counterproductive – for cells to produce proteins not appropriate for the cell’s function. Gene regulation refers to the mechanisms by which cells control gene expression.
The presence or absence of a protein, as well as the amount of protein produced, affects cell function. For example, in humans and other mammals, red blood cells transport oxygen throughout the bloodstream. Red blood cells contain large amounts of hemoglobin, a protein which binds oxygen in the lungs and releases it in oxygen-poor tissues. Hemoglobin is not expressed in other cells. B cells are specialized white blood cells that participate in the immune system. B cells produce antibodies, which are proteins used to launch an immune response against pathogens like viruses or bacteria. Antibodies are not produced by other cell types not involved in the antibody-mediated immune response.
On the other hand, some proteins, called housekeeping proteins, are needed in all cell types. For example, in both eukaryotes and prokaryotes nearly all cells require the enzymes used in glycolysis, the metabolic pathway that couples the breakdown of glucose to the production of ATP. Housekeeping genes like those of glycolysis – fundamental to the function of the cell – are always active.
Even a single-celled organism will not express every gene all at once. RNA and protein production are energetically demanding, so genes that are not needed will not be transcribed or translated. For example, sometimes glucose is not available as a food source, and cells would need to use a different set of enzymes to break down alternative sugars. Those enzymes are only needed sometimes, so the genes are only expressed under those specific conditions. Likewise, genes required to synthesize certain amino acids are only active when the cell is short of those amino acids. Genes involved in stress response are only active when the cell is stressed.
This module begins with an overview of the mechanisms of gene regulation, with additional focus on regulation at the level of transcription. We discuss the action of transcriptional activators and repressors, including how multiple genes can be co-regulated to coordinate expression. Finally, this module ends with three well-studied examples of gene regulation in bacteria: the lac operon illustrates how activators and repressors can work together to regulate a gene, the lambda repressor demonstrates how a single protein factor can act as both an activator and a repressor, and the trp attenuator shows how, in prokaryotes, the process of translation can affect whether or not a gene is transcribed.
Media Attributions
- Genome, transcriptome, proteome © Amanda Simons is licensed under a CC BY-SA (Attribution ShareAlike) license